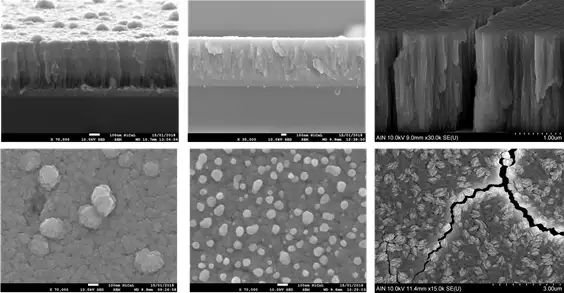
By Dermot Monaghan, Victor Bellido-Gonzalez, Tommaso Sgrilli, Patrick McCarthy and Lara Maroto Diaz of Gencoa, Ltd., and Oihane Hernandez-Rodriguez of Fundación Tekniker, Eibar, Spain
A novel human coronavirus that now is named severe acute respiratory syndrome coronavirus 2 (SARS-CoV-2 – formerly called HCoV-19) emerged in Wuhan, China, in late 2019 and now is causing the COVID-19 global pandemic. Until widespread vaccination, there is a strategic need to invest in technological solutions to reduce and slow the impact of the pandemic. The SARS-CoV-2 virus can be present on plastic and glass surfaces for several days. Once a hand has touched a contaminated surface, hand-washing reduces the probability of infection by removing the virus. Gloves reduce the chance of contagion if changed frequently, although the virus will be transferred by a gloved hand to the next surface. Once travel restrictions are relaxed, the chance of spread from surfaces will increase as the population begins to move around again. Vacuum coating has a solution that can be used to greatly reduce the spread from commonly touched surfaces. Vacuum-deposited, copper-based, nanostructured films are highly biocidal and create self-sanitizing surfaces. These films have a viral- and microbial-killing effectiveness above conventional cleaning methods. The killing action is continuous; hence it will have a positive impact to reduce the probability of cross-contamination from surfaces. Tests show complete killing of 1 million coronavirus particles placed on such surfaces within 15 mins.
This paper presents a solution against all forms of infection derived from touch surfaces by using a nanotechnology coating-based adhesive pad to protect the public from cross-contamination. The coating development has been focused on flexible and transparent substrates which easily can be upscaled using roll-to-roll (R2R) vacuum-production techniques. Different biochemical and biological tests have been performed to quantify the biocidal activity.
Editor’s Note: This paper is based on a presentation at the 2020 AIMCAL R2R USA / SPE FlexPackCon® Virtual Conference, held online in October 2020.
Introduction
The 2019 SARS-CoV-2 outbreak responsible for the initial COVID-19 epidemic has presented an unprecedentedly high velocity of virus contagion. While the virus can be destroyed by hand-washing with appropriate disinfectants, surfaces, screens and mobile phones, once touched, can re-contaminate the user and pose a biothreat risk for infection spread. They can contribute to the disease crossing borders especially as they are omnipresent in modern transport and human-to-human, social-contact scenarios. Screens and other surfaces also can contribute to the contamination and genesis of additional secondary fomites (doorknobs, airport self-check-in stations, bus rails, ATM monitors, lift buttons, etc.). Evidence from a Wenzhou shopping mall in China indicates that fomite transmission on surfaces fueled the outbreak [1]. Such indirect transmission is a result of the virus being present on surfaces with which an infected individual has interacted. A study by the University of Nebraska Medical School treating patients from the Diamond Princess Cruise Ship showed 72.4% of surfaces near to patients were positive for the viral RNA and 77.8% of cellphones were contaminated [2].
Evidence in a letter published in the New England Journal of Medicine on March 17, 2020 [3], finds that the stability of the virus SARS-CoV-2 is “similar to that of SARS-CoV-1 under the experimental circumstances tested.” The new coronavirus was found to be viable up to 72 hrs after being placed on stainless steel and plastic. It was viable up to 4 hrs after being placed on copper and up to 24 hrs after being put on paperboard. On copper, no viable SARS-CoV-2 was measured after 4 hrs and, importantly, the decay of the virus is exponential. Hence the dose of SARS-CoV-2 that is likely to be received from a biocidal surface is much lower than comparable materials, and this is important information for pandemic-mitigation efforts. Previous work by Keevil at Southampton University [4] in the UK also has shown that a relatively low concentration of enveloped respiratory viruses may retain infectivity on common hard surfaces for longer than previously thought (5 days) and copper surfaces were shown to disable a high proportion of coronavirus within 5 mins and entirely within 30 mins.
All forms of surfaces present a contagion risk, whether it be from a viral or microbial source, but the widespread use of touchscreens in healthcare and community settings is a possible “Trojan Horse” contributing to the transmission of microbial infections in epidemics and pandemics [5].
Touchscreen technology is seen as “safer” than face-to-face contact. Supermarket self-checkouts are a good example, with a constant flow of use and interaction from both staff and customers. Another is a doctor’s surgery, where sick people all are asked to touch the same surface in a steady flow.
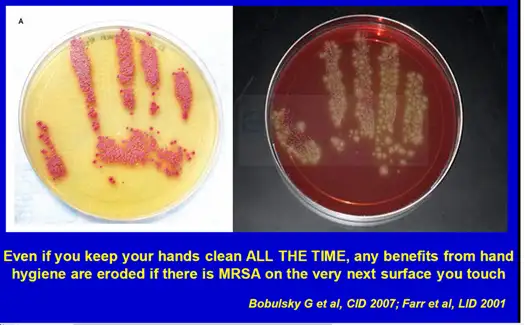
Viral contamination certainly will remain present on a touchscreen for hours and days if not removed by cleaning (see Figure 1). There is an urgent need for a smart means to self-sanitize surfaces to reduce contagion risk. The desirability of this approach also is backed-up by the inability of conventional cleaning methods to effectively sanitize surfaces. This is evident from the high levels of Healthcare-Associated Infections (HCAI), as well as studies of contamination on surfaces found within medical settings [6].
This article presents a solution against infections derived from touch surfaces by using a nanotechnology-based, vacuum-deposited, coated adhesive pad to protect the public from cross-contamination. The coating development has been focused on flexible and transparent substrates and coatings which easily could be upscaled using R2R-production techniques. Different biochemical and biological tests have been performed to quantify the biocidal activity.
Before the COVID-19 pandemic, Healthcare-Associated Infections (HCAI) were the prime focus for self-sanitizing, surface-technology developments. HCAI poses a serious risk to patients as they can result in significant harm to those infected.
The estimated HCAI incidence rate in the USA (2014) was 4.5%, corresponding to 9.3 infections per 1,000 patient-days and 1.7 million affected patients [6]. The European Centre for Disease Prevention and Control (ECDC) estimated that 4,131,000 patients are affected by approximately 4,544,100 episodes of HCAI every year in Europe [6].
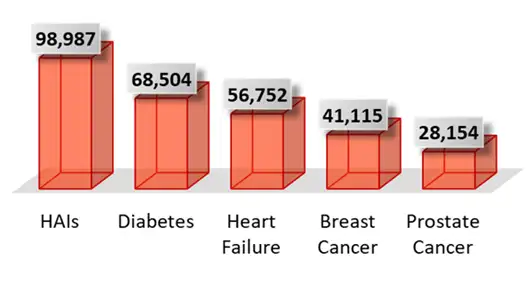
The Agency for Healthcare Research and Quality reported that HCAIs are the most common complications of hospital care and one of the top 10 leading causes of death in the USA (see Figure 2) [7].
Reducing healthcare-associated infections (HCAIs) remains a key indicator for most developed countries when measuring public safety and quality of care.
The main objective of this work is the creation by vacuum coating of highly biocidal surfaces that rapidly will kill viral and microbial strains.
Copper as a biocidal material
Copper materials have been used since ancient times for their antimicrobial properties, and modern science has demonstrated how incredibly effective such a surface is in terms of bacterial and viral killing. Technology based upon bulk copper materials now is available that can minimize the survival of germs on surfaces; the main barriers remain cost and convenience.
Studies have shown that copper surfaces, for instance, have a LOG 3 (99.9%) effectiveness in killing microbes as copper-metal ions are highly efficient in this regard. This is superior to rigorous chemical cleaning. The cost, however, of manufacturing and replacing fixtures and fittings with copper components has prevented widespread uptake despite clear evidence of benefits.
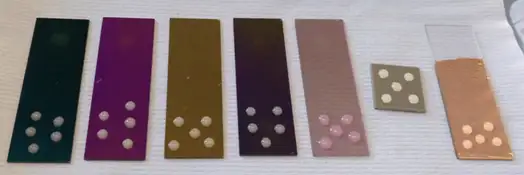
The background to creating biocidal coatings is based upon two strands of wider research. The first is the use of metallic surfaces as effective antimicrobial agents. The second is the role of the nano-scale topography of a surface to provide enhanced antimicrobial performance. Extensive work on Ag, Cu, Ti and Zn materials has illustrated their use as antimicrobial agents. Likewise, work on nanostructured surfaces and nanoparticles has yielded good results for antimicrobial performance. Nano-structuring of surfaces alone can produce environments that effectively kill microbes on a LOG 2 to 4 scale (99 to 99.99%). If this nano-structuring is combined with a metal-ion leaching effect, metallic-based nanostructured materials have the potential to provide highly effective antimicrobial and, consequently, antiviral surfaces.
Vacuum deposition is used industrially to deposit thin layers of virtually any material type onto all forms of surfaces. Certain vacuum-deposited layers can combine several biocidal actions: the presence of metal ions, and the creation of a nano-topography that promotes chemical and electrical activity between features [8]. Prior work by Gencoa has shown that these combined effects are required to produce the highest levels of antimicrobial effectiveness [9,10]. Under the correct deposition conditions, a range of metals can result in a microbial kill rate of LOG 6 (99.9999%) within minutes. In terms of antimicrobial performance, these layers perform as well as the best nano-clustered silver surfaces.
Over the last six years, Gencoa has created coatings with varying degrees of complexity for direct application onto 3D objects. Such coatings can be tailored to provide extreme hardness, low friction, different color hues or even transparency. So, vacuum-deposition provides the coating “armory” to create the critical coating-microstructure required to enhance biocidal performance of these materials.
To more rapidly exploit the technology and impact the battle against the SARS-CoV-2 virus, the recent focus is to create 5- to 100-nm-thick layers based upon copper on top of a thin plastic web, which then can be adhered onto a touch surface. The choice of copper-based layers is a “safe” approach because copper and a wide range of its alloys are the only materials approved by the US Food & Drug Administration (FDA) to make public claims of antimicrobial activity. All other materials, including silver, have failed to gain approval. Copper is lower cost than silver and can be sputtered at high rates compared to other metals.
Although oversimplified, in a practical sense, the concept is a copper version of an aluminum-metallized “snack-food” package. Importantly, it is a very low-cost manufacturing process, and suitable surfaces simply can be covered by the adhesive film to afford months and years of protection, which avoids costly hardware changes. Flat surfaces easily are protected, as are simple-geometry 3D shapes. More complicated shapes can be protected by heat-shrinkable, coated plastic or have a physical vapor deposition (PVD) coating applied directly.
There are two market requirements of equal importance: touch surfaces and touchscreens. Hence, both opaque and transparent forms of coating on plastic are needed to protect the existing built environment. Opaque forms are more readily produced as they can be simple copper or copper-alloy films. Transparent forms present more of a challenge, and a new form is presented here that has great potential for exploitation.
Coating deposition and bioactivity
A physical vapor deposition (PVD) method based upon magnetron sputtering was used to produce the different coatings on surfaces, as described generically in reference [11]. The PVD unit to create the layers comprised a vacuum vessel (1600 L/s turbopump), two off Gencoa magnetron-sputtering sources and one off Gencoa linear-ion source. During coating, the substrates were rotated in front of the plasma sources. The substrates were attached to a suitable substrate holder.
To create an effective coating that quickly destroys organic matter on contact, a number of features need to be combined. The first is the correct growth structure and corresponding nano-topography. Sputter deposition has the ability to create very dense and featureless coatings. For many applications, this is the aim, and the coating process is tuned to create smooth layers. However, when creating a hostile environment for microbes, bacteria and viruses, smooth surfaces are to be avoided.
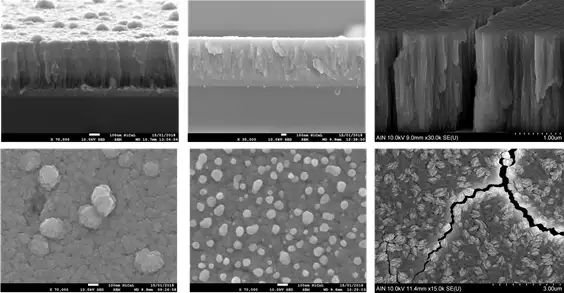
If the growth features of vacuum-deposited layers have some degree of “separation” to their neighbors, the separation will provide a subtle difference in electrochemistry. Once the growth features are bridged by an organic body, it creates a highly effective mechanism to disturb and kill the microbe or virus. While there also are surface-area differences between smooth and rougher sputtered layers, this alone does not explain why pure copper transitions from very low to highly biocidal as the nano-topography changes.
Figure 4 illustrates different sputtered surface coatings with varying separation between features. All these layers provide high levels of biocidal activity, and only the left images contain some copper elements. Not all material types can create the same activity – some clearly are more effective than others. Hence, the role of metal-ion leaching, toxicity and reactive-oxygen species generation will determine the effectiveness of any final coating structure.
The two main characteristics that produce an effective biocidal coating are: 1) The chemical nature of the surface (the coating material); and 2) the topography of the surface (growth structure).
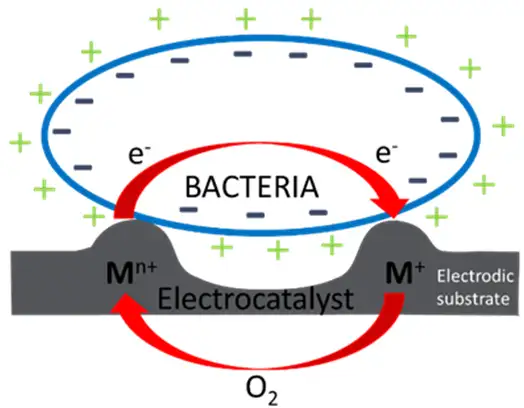
Regarding the chemical nature of the material, it is known that copper can interact with lipids, causing their peroxidation and opening of holes in the cell membranes, thereby compromising the integrity of cells [12].
One additional factor for the biocidal efficiency of coatings is related to topography. The most efficient biocidal coatings benefit from coating features that enhance the oxidative electrocatalytic process [13] (see Figure 5).
The generation of reactive-oxygen species is widely cited as an important mechanism for surface bioactivity. Driving this reaction requires different surface features to promote electrical potential differences which are bridged by the microorganism. Hence, creating a coating that has distinct growth structures is an important aspect of any biocidal, vacuum-deposited layer. These nanofeatures easily can be created by the vacuum-coating method, which sets it apart from other means of structuring surfaces.
Part 2 of this paper will cover production of copper-based and transparent coatings, the biochemical method for assessment of biocidal activity of vacuum-deposited coatings and biocidal results of sputtered transparent layers.
Acknowledgements
The authors would like to thank Joseba Esparza-Gorraiz, José Fernández-Palacios and Gonzalo Garcia-Fuentes of AIN Surface Technology Group (Asociación de la Industria Navarra, Spain, www.ain.es/tecnologia/superficies/) for their assistance with the antimicrobial JIS Z 2801:2000 and viral test. The Open Innovation Hub for Antimicrobial Surfaces at the University of Liverpool, UK. Innovate UK project 103820 (2017), 78311, 85336, 85562 (2020). Erasmus Project nº 2019-1-ES01-KA103-063357.
References
- EID Journal, Vol. 26, No. 6—June 2020, Research Letter, “Indirect Virus Transmission in Cluster of COVID-19 Cases,” Wenzhou, China, 2020. Jing Cai, Wenjie Sun, Jianping Huang, Michelle Gamber, Jing Wu, and Guiqing He.
- “Aerosol and Surface Transmission Potential of SARS-CoV-2,” Joshua L Santarpia, et al. COVID-19 SARS-CoV-2 preprints from medRxiv and bioRxiv.
- “Aerosol and Surface Stability of SARS-CoV-2 as Aerosol and Surface Stability of SARS-CoV-2 as Compared with SARS-CoV-1.” April 16, 2020. New England Journal of Medicine, 2020; 382:1564-1567
- “Human Coronavirus 229E Remains Infectious on Common Touch Surface Materials,” Sarah L. Warnes, Zoë R. Little, C. William Keevil, Journal of Hospital Infection, Vol. 99, Issue 3, July 2018, pp 239-249.
- Goldblatt J.G., Krief I., Klonsky T., Haller D., Milloul V., Sixsmith D.M. “Use of cellular telephones and transmission of pathogens by medical staff in New York and Israel.” Infect Control Hosp Epidemiol. 2007; 28:500–503. doi: 10.1086/513446; Travel Med Infect Dis. 2020 Apr 28: 101704. doi: 10.1016/j.tmaid.2020.101704 [Epub ahead of print]
- B. Allegranzi, S. Bagheri Nejad, C. Combescure, W. Graafmans, H. Attar, L. Donaldson, Prof., D. Pittet, “Burden of endemic health-care-associated infection in developing countries: systematic review and meta-analysis,” The Lancet, Dec. 10, 2010. https://doi.org/10.1016/S0140-6736(10)61458-4
- M. Heron, et al. “Deaths: Leading Causes for 2009.” National Vital Statistics Reports 2012, Vol. 61, No.7, Agency for Healthcare Research and Quality [webpage on the Internet] Patient safety primers: healthcare-associated infections. 2012. [Accessed April 3, 2018].
- “Bio Control Activity Surface,” EP 2981501, US 10617120 (2016-02-10).
- “High-Efficiency Antimicrobial Coatings for Biomedical Applications.” Authors: Oihane Hernandez-Rodriguez, Victor Bellido-Gonzalez, Dermot Monaghan Published: 2018 Society of Vacuum Coaters TechCon.
- “Antimicrobial Coatings for Touch Surfaces.” Authors: Oihane Hernandez-Rodriguez, Dermot Monaghan, Victor Bellido-Gonzalez. Published: 2019 Society of Vacuum Coaters TechCon.
- D.M. Mattox, and V.H. Mattox, ed., 50 Years of Vacuum Coating Technology and the Growth of the Society of Vacuum Coaters, Society of Vacuum Coaters, 2007.
- Adney, B., & Baker, J. (1996). National Renewable Energy Laboratory. Technical Report NREL/TP-510-42628, http://www.nrel.gov/biomass/pdfs/42628.pdf
- “Copper-induced formation of reactive-oxygen species causes cell death and disruption of calcium homeostasis in trout hepatocytes.” Manzl, C, Enrich, J, Ebner, H, Dallinger, R, Krumschnabel, G (2004). Toxicology 196 (1–2): 57–64.
Dr. Dermot Monaghan of Gencoa, Ltd. (Liverpool, UK), can be reached at +44-151-486-4466, direct: +44-151-541-6030, email: [email protected], www.gencoa.com.
Oihane Hernandez-Rodriguez of Fundación Tekniker (Eibar, Spain), can be reached at +34-943-20-67-44, https://www.tekniker.es/en.