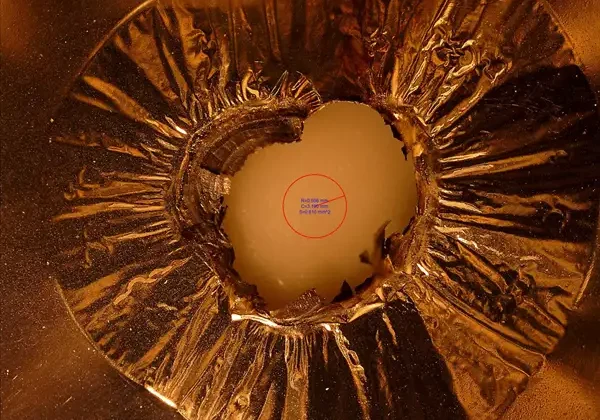
By Dirk Van Hyning, Ph.D., chief consortium officer, Soteria Battery Innovation Group
Abstract
In the $5-billion lithium-ion battery (LiB) industry, which is growing at 22% per year, fires due to “thermal runaway” are a costly, deadly and growing issue. Innovative new materials have been developed by this firm to interrupt thermal runaway, one of which is a metallized-plastic film to replace the conventional metal-foil current collector. This technology makes the batteries demonstrably safer while also reducing weight and cost. We are bringing these technologies to market through a 54-member (and growing) global LiB-industry consortium – which includes NASA, Mercedes, BOSCH, Motorola, Dupont and Teijin, among others.
Introduction
On the evening of March 10, 2017, just before 8 p.m. in the town of Harrisburg, PA, a fire broke out on the first floor in the Lexington Street home of Chaka Crawford and Steven Dominick. By the time the event was over, 2-year-old Ashanti Hughes, 10-yr-old Savannah Dominick and firefighter Dennis DeVoe were dead. The cause of the fire: exploding lithium-ion batteries (LiB) inside a Hoverboard toy, which, at the time, rapidly was growing in popularity. Before the Hoverboards eventually were recalled, the Consumer Product Safety Commission (CPSC) reports that there were over 165 fire incidents in 38 states and an estimated 10,000+ emergency room visits due to faulty lithium-ion batteries within Hoverboards [1].
Unfortunately, the issue is not limited to just Hoverboards but endemic to the materials and basic architecture of the highly energetic LiB cells. CPSC data show that between 2012 and 2017, there were 4.2 million products recalled in 49 separate recalls in product categories ranging from laptops to power banks to cellphones [1]. The same data also show over 21,000 incident reports in 483 product categories, showing a staggering breadth to the issue.
Today, the problem is growing in scale, as electric vehicles and buses are shifting the landscape of where the majority of LiBs (or “cells”) are being used. Whereas a Hoverboard, which caused the fatal Harrisburg fire, might contain 20 Type 18650 cells (an 18650 is a common, cylindrical-LiB cell type similar in appearance to a large AA battery), a common electric vehicle (EV), such as a Tesla Model S, contains more than 8,000.
The growing popularity of EVs, coupled with the sheer volume of LiB cells required for each vehicle, is the reason why the global consumption of LiB cells is forecasted to increase from roughly 125 GWh (gigawatt hours, a common measure of LiB consumption) in 2017 to nearly 300 GWh this year, with sustained annual growth exceeding 20% [2]. Given the potentially crippling cost of a product recall (for example, the LiB-driven 2016 Galaxy Note 7 recall and discontinuation cost Samsung an estimated $17 billion dollars [3]), the entire LiB industry is pursuing technologies intensely to minimize and/or eliminate these costly and deadly fires.
Architecture of an inherent issue
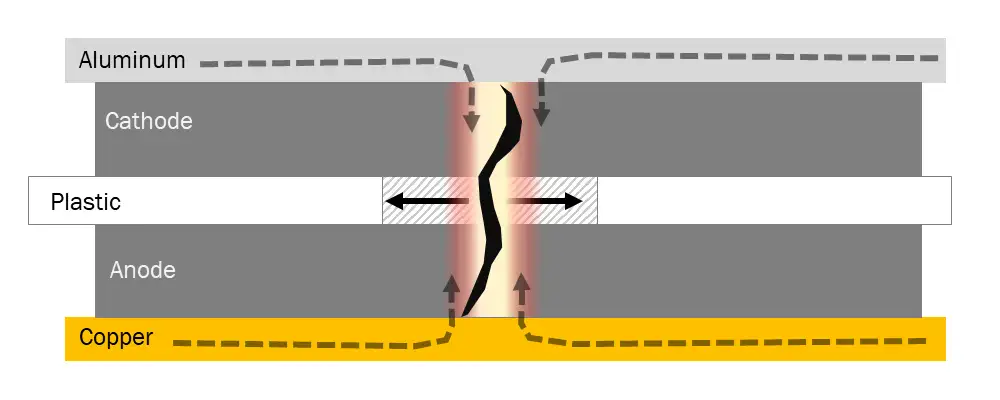
The basic architecture of LiBs, regardless of capacity or form factor, essentially is identical. On one side, there is an anode material, and on the opposite side a cathode material, with a thin separator between the two sides preventing physical contact. Under electrical load, electrons flow from one side to the other through metallic-foil current collectors while an organic electrolyte allows lithium ions to flow from the cathode to the anode to maintain electrical balance within the system. The critical component in virtually all LiB-fire events is that organic electrolyte. The electrolyte typically consists of lithium salts dissolved in organic carbonates, which, when heated above a critical temperature, ignite and expand with intense flame and heat, with temperatures quickly approaching 1,300° F.
Several events can cause a battery to catch fire or go into “thermal runaway” – an industry term for fire – such as ambient heat or an attempt to charge or discharge the cell too rapidly, but the most common is an electrical short that develops within the battery. Very similar to an electrical short inside a house, a battery short can occur when the battery is damaged, (i.e., an EV crashes or a cellphone is bent), but the more common cause of shorts, and the most worrisome, is due to what are called “latent defects.” Latent defects are objects, such as small metal particles or poorly ground electrode material, introduced into the cell during manufacturing that work their way into and through the separator as the battery is used and goes through expansion/contraction cycles.
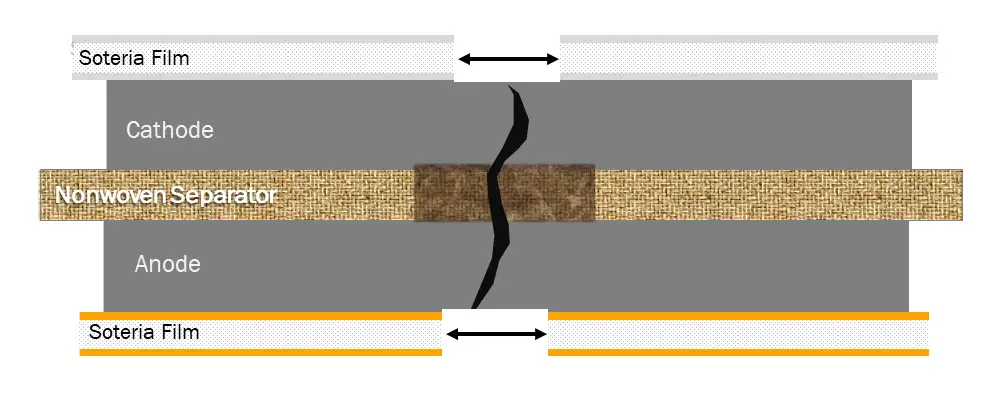
When such a short occurs (see Figure 1a), the robust metal foil current collectors begin to dump all of the electrical energy of the cell into the short, resulting in very high currents through the short and thus rapidly increasing localized temperatures. As the temperature reaches a critical level (typically 100° to 130° C), typical plastic separators begin to melt and retreat from the short, which in turn drives more current into the short, and a vicious cycle ensues (thus the term “thermal runaway”), resulting in the flammable electrolyte catching fire.
Making the strong weak and the weak strong
Soteria’s approach is to interrupt this basic mechanism of thermal runaway by reversing the dynamic of how the separator and current collectors respond to a temperature rise during a short. The first of our two technologies (see Figure 1b) is a nonwoven-material separator made from commercially available, specialty grades of polyester, lyocell and aramid fibers. Figure 2 shows the physical deformation (i.e., strain) of three types of separator materials (polyolefin, ceramic-coated polyolefin and this firm’s novel separator) with increasing temperature. Even with ceramic coating, polyolefin-based separators shrink catastrophically at around 125° to 150° C. The novel nonwoven separator, due to the thermal properties of the aramid and the charring behavior of the cotton, is thermally stable beyond 300° C, a temperature at which the electrolyte already would have ignited. In short, the new separator does not aggravate a short at the temperatures critical to initiation of thermal runaway.
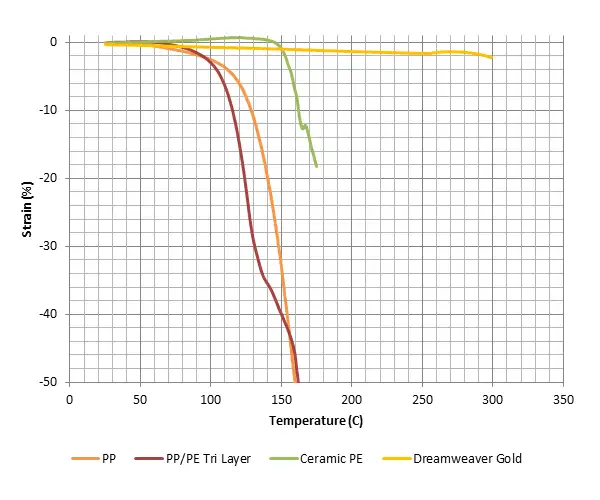
However, for more energetic cells, the original short often can generate enough heat, even with a stable separator, to send the LiB cell into thermal runaway. The second technology addresses the flow of current into the short but replaces the metal-foil current collectors. The metal foils that carry the electrons from one side of the cell to the other are roughly 10-15 microns thick, very similar to consumer-kitchen aluminum foil. This foil is limited to a minimum 10-15 microns – not because the cell needs that amount of metal to function, but because thinner foils are too mechanically weak to survive the high-speed coating and winding processes necessary to incorporate them into cells. With the exception of cells that require high power (think power tools), only about 10% of the metal in a current collector is needed to serve the day-to-day function of the cell.
Our development is to replace that robust metal foil with a thin (3 to 8 micron) polymer film and metallize both sides with 500 nm of metal. Figure 3 shows how the new current collectors respond to a short. When a short develops (size of red circle) and high currents cause a spike in temperature, at a critical temperature long before the electrolyte ignites, the thin metal layer oxidizes and the underlying polymer film melts and retreats from the short, thereby isolating it from current flow. In essence, the new metallized-plastic, film-based current collector acts as an internal fuse, resulting in a small “dead spot” in the current collector but otherwise leaving a fully functioning cell.
Improved safety performance
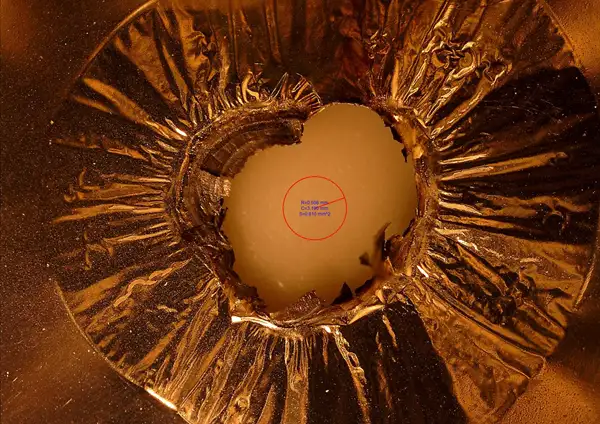
The ultimate test of any technology, safety or otherwise, is how it performs in real-world situations and in actual products. For this firm’s new technologies, that means putting our materials in cells that the industry uses, made by established industry manufacturers. LiBs come in a wide range of form factors and properties. Over the past two years, we have (with new builds happening continually) made cells in most major form factors, with most of the widely used cathode chemistries for applications aimed at everything from space flight to automobiles to wearable military batteries.
With very little exception, replacing conventional materials with these new metallized-plastic film current collectors and nonwoven-material separators, in varying combinations, result in unprecedented safety performance. Figure 4 shows a static image of a nail penetration test (taken from a high-speed X-ray video)
of the cross-section of cylindrical Type 18650 cells built by NASA. Nail penetration is one of the most difficult tests of a cell’s safety as it is used to simulate catastrophic damage. The top image of Figure 4 is a cell made with conventional materials, showing the contents in severe disarray because that cell has
gone into thermal runaway and is ejecting its contents out of the top of the cell. This happens with such certainty that every end user (i.e., EVs, energy-storage systems, etc.) designs the safety system of their battery packs assuming this will occur. The bottom image is the identical cell with only the aluminum foil current collector replaced with our new metallized-film development. Not only does the cell not react at all to insertion of the nail, the cell still functions up to weeks later. This behavior is not only virtually without precedent but also is of critical importance to industries such as battery-powered aircraft, for obvious reasons.
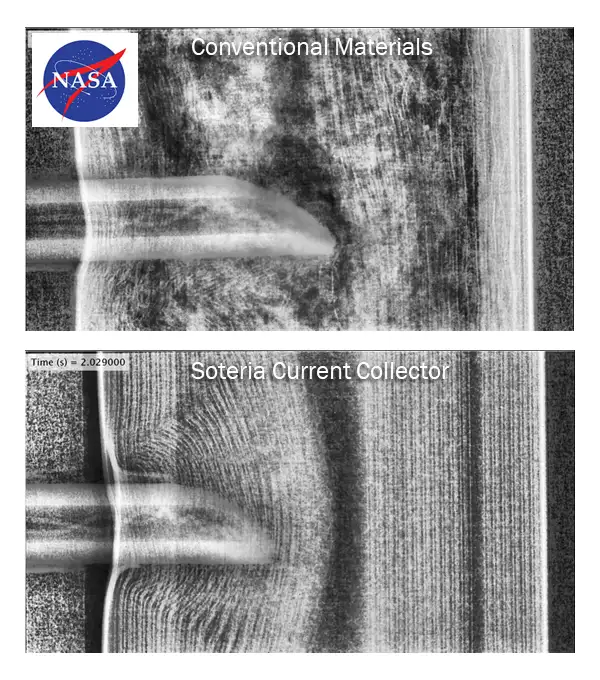
Almost as important to widespread adoption as the safety performance, these new technologies achieve this result by removing expensive metal and replacing it with inexpensive polymer, thereby reducing the cost as well as the weight. With continued success, this firm may be able to actually put end users in the position of having to pay more to get a heavier, more dangerous battery.
Using Game Theory to save lives
During the last 10 years, there has been a steady and growing stream of new technology companies aimed at the LiB industry. However, several realities about this industry have resulted in most of those companies ultimately failing. First, like a lot of other industries, for a cell company to leverage purchasing power, rarely, if ever, will it agree to adopt any component that will be sole-sourced, much less a critical component like a separator or current collector. LiB companies demand options. Second, poor material quality in the components of LiB cells can have catastrophic results, such as the $17-billion Samsung Galaxy Note 7 recall, the Boeing 787 groundings or – worst of all – deaths like the Harrisburg Hoverboard fire. As a result, not only does the LiB industry demand options, but it demands world-class, global providers of the highest quality materials. These two market forces have proven to be insurmountable for the vast majority of new technology companies using a traditional “go it alone” business model.
However, there are myriad examples of new technologies being widely adopted in equally demanding industries: Blu-Ray, HDTV and Bluetooth, just to name a few. While each of these technology launches have different details, they all rely on one key concept: coopetition. Coopetition (the combination or “cooperation” and “competition,” a modern rebranding of Game Theory[4]), is the concept of collaboration between business competitors in the hopes of mutually beneficial results. In the case of the LiB industry, those “beneficial results” are 1) overcoming the two key hurdles to market entry discussed above and 2) growing the entire market opportunity by making LiB cells safer.
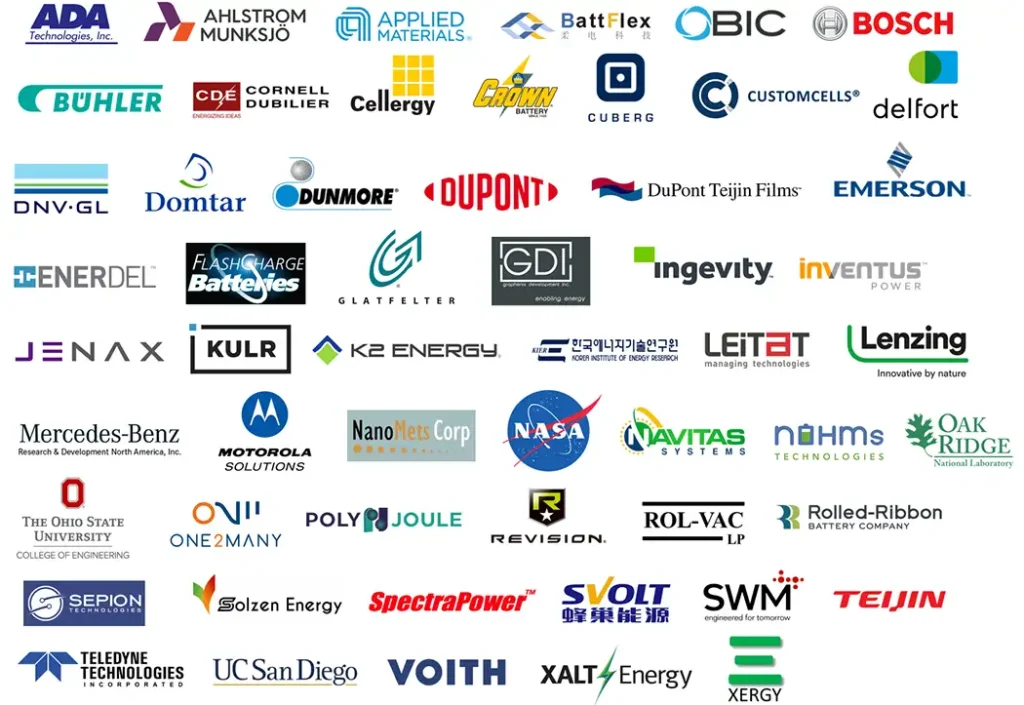
This firm’s path-to-market borrows heavily from one of the most successful implementations of the open-innovation “coopetition” model – Bluetooth. In the Bluetooth/Soteria model, the basic approach is to form a group of companies from throughout the global LiB industry and all work together to collectively advance the technology, license the technology to a small group (~5) of global, world-class material producers and then develop a highly aggressive safety standard to drive adoption. Figure 5 shows the current Soteria Consortium as of press time. It has quickly grown to 54 members since its inception two years ago. The membership contains four general types of companies:
- End users and battery/cell producers: these are the companies who need safer cells and ultimately drive adoption
- Separator and current collector producers: these are vacuum-metallizers and papermakers that ultimately license the technology and produce material
- Material suppliers to the licensees: this group includes film and fiber producers, as well as equipment manufacturers
- Lastly and very importantly are other small technology companies that also bring safety technologies to the mission and see the consortium as a means to get their technologies adopted without having to bear the huge burden of doing all the work themselves.
A massive opportunity
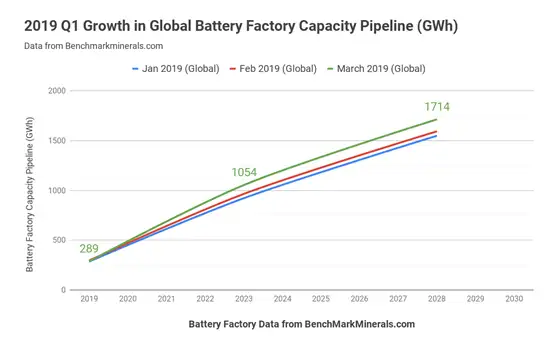
For the first 15 years of the existence of LiBs (1997-2012), the vast majority of cells were used in small electronics (i.e., power tools and cellphones [5]). In that time, the total consumption of LiBs went from zero to approximately 35 GWh. Since the development of electric vehicles and rechargeable-battery energy-storage system (ESSs) over the last seven years, the fraction of cells consumed outside portable electronics shifted from ~5% in 2012 to over 85% by 2019 [5]. In addition, the sheer number of cells needed for EVs and ESSs has resulted in the global consumption increasing from 35 GWh in 2012 to over 289 GWh last year, and announced capacity increases will result in 1,714 GWh annual consumption by 2028 [2] (see Figure 6).
This level of cell consumption translates into an annual need for approximately 17.1 billion sq meters of separator material and 8.55 billion sq meters of both copper and aluminum current collector material by 2028. At current average market prices of conventional separator and metal-foil materials ($1.00 for separators, $0.65 for Al current collector and $2.70 for Cu current collector), this consumption represents a roughly $17-billion market for separators, $5.6 billion for aluminum current collectors and $23 billion for copper current collectors, with significant upside if EV and ESS adoption happens more rapidly than estimates show.
Given our goal of having a limited number of licensees (~5) of each technology worldwide, even a modest penetration of this market presents the opportunity for significant, high-margin revenue streams. However, this firm believes, and the market has reinforced the belief, that significant penetration can be possible only if there is healthy “coopetition” that the consortium provides and results in multiple, world-class supply options.
Conclusion
Progress toward the mission of ending thermal runaway in LiBs has been extremely strong over the last several years. The consortium has grown from nothing to over 50 members. Multiple consortium members now can provide high-quality separators and current collectors. Full commercial-scale members have produced a number of real-world cell builds showing radically improved safety, and consortium membership now has a global footprint. The work, however, is far from over.
Electric vehicles will need to go farther, and power tools will need to run longer. Vehicles, aircraft and personal devices will be designed with highly energetic cells closer and closer to humans in confined spaces. Demands will be placed on batteries to pack more and more energy into the same space. This firm’s separator and current-collector technologies increasingly prove to be a powerful approach to these challenges, but they won’t be the only approach. As Soteria member companies continue to advance the core technologies and the consortium continues to grow, the mission will remain the same: End thermal runaway by using any technology necessary, partnering with any company who can advance the effort and supporting any organization with the same goal, whether they be consortium members
or competitors.
Consortium companies are bound by the belief that if we stay true to our mission and not just to ourselves, never again will any family have to suffer like those of Ashanti Hughes, Savannah Dominick and Dennis DeVoe, who died in that fateful 2017 Harrisburg fire. The power to do that is now within our grasp.
References
- US Consumer Product Safety Commission: Lithium-Ion Battery Safety Standards for Consumer Product Import into the United States from https://www.cpsc.gov/s3fs-public/3LeeCPSC.En_.pdf?QMvz78vcq0web.KaXE_TJD.dpk7DbADF
- 2019 Q1 Growth in Global Battery Factory Capacity Pipeline from http://www.benchmarkmaterials.com
- https://www.reuters.com/article/us-samsung-elec-smartphones-costs-idUSKCN12B0FX
- Journal of Applied Economic Sciences 9(3 (29)):458-469, January 2014
- The Rechargeable Battery Market and Main Trends 2017-2025, Avicenne Energy, 2018
Dirk Van Hyning, Ph.D., chief consortium officer at Soteria Battery Innovation Group (Greenville, SC), spent 15 years at Milliken & Co., one of the largest private textile companies in the world. His last role there before joining Soteria was as CEO and managing director of Alexium, Inc, a publicly listed chemical company on the Australian Stock Exchange. Van Hyning holds a Bachelor of Science in Chemical Engineering from North Carolina State University, and Master’s of Science and Ph.D. degrees from the University of Illinois at Urbana-Champaign. He can be reached at 864-216-5230, email: [email protected], www.soteriabig.com.