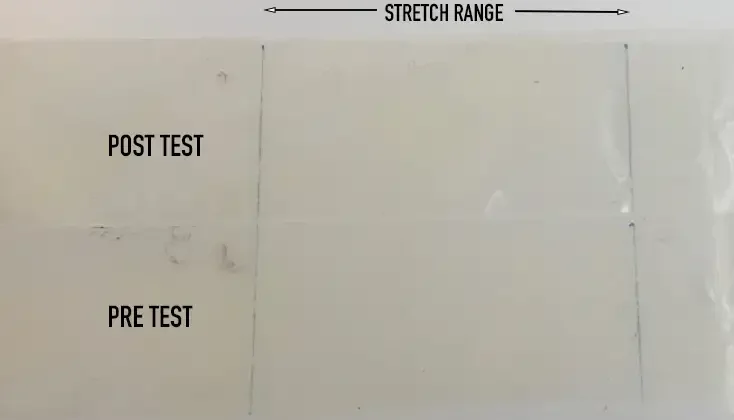
By Andy Behr, technology mgr.-Panasonic Electronic Materials Division
A primary factor limiting the deployment of printed electronics is a dearth of application-specific substrates. Conventional thermoplastic polymer films like TPU and PET, typically employed for printed electronics, exhibit poor temperature resistance and relatively high hysteresis, creating significant challenges for printing and curing of functional materials, such as conductive pastes, surface-mount assembly processes and end-use durability. Researchers at this firm developed a new class of thermoset: soft, high-temperature resistant, stretchable film designed specifically for printed electronics and soft circuit manufacturing. This article will discuss the substrate technology in detail, including testing results and highlight some use-case parts fabricated with these materials.
Introduction
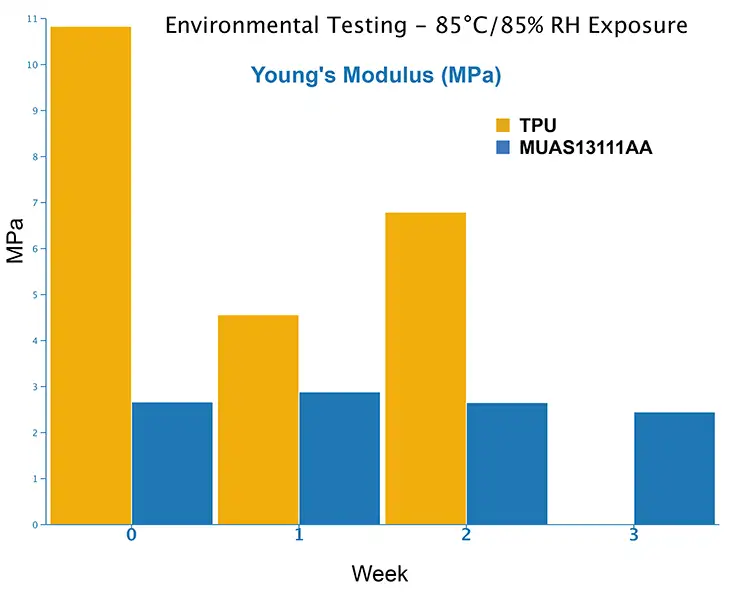
One of the primary factors limiting the deployment of printed electronics is a dearth of application-specific substrates. Conventional and readily available thermoplastic polymer films, such as thermoplastic polyurethane (TPU) and polyethylene terephthalate (PET), historically have been pressed into service for making printed-electronics circuits. However, these materials typically exhibit poor temperature resistance and relatively high permanent deformation after strain (hysteresis), creating significant challenges for the printing and curing of functional materials: conductive pastes; surface-mount assembly processes; and end-use durability. Researchers at this firm have developed a new class of thermoset films which are soft, highly temperature-resistant and stretchable – designed specifically for printed electronics and soft circuit manufacturing.
What ARE printed electronics?
Of late, printed electronics have come to the fore of the electronic-device zeitgeist along with other emerging technologies such as Extended Reality/Virtual Reality/Mixed Reality, Artificial Intelligence/Machine Learning, 6G, IoT, Quantum Computing and the like. Therefore, it makes sense to define the technology prior to delving into the details.
IPC (formerly the Institute of Printed Circuits) is an industry trade association whose aim is to standardize the assembly and production requirements of printed circuit boards (PCBs). According to IPC-T-51, Terms and Definitions for the Manufacture of Printed Electronics, Printed Electronics are devices or components manufactured by the deposition of functional materials using printing processes (screen printing, gravure, flexographic, jetting, etc.) [1].
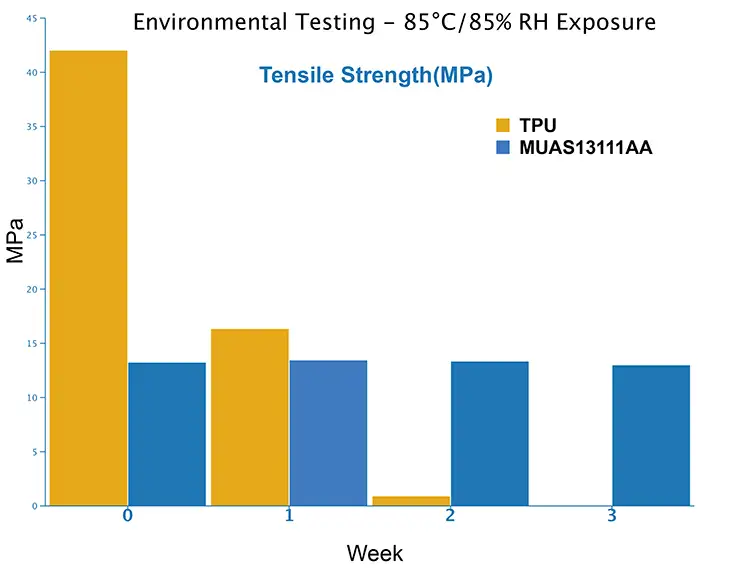
Perhaps a bit circular in nature, but this definition serves to capture the diverse breadth of manufacturing processes collected under the printed-electronics umbrella. Additionally, it juxtaposes the additive nature of printed electronics from the (primarily) subtractive method of forming conductive structures in traditional PCB manufacturing.
While printed electronics is useful and a well-recognized moniker, an entire panoply of newly minted and recently resurrected terms are employed to describe similar or adjacent technologies, including Flexible Hybrid Electronics (FHE)[2], Stretchable Hybrid Electronics (SHE) [3], Stretchtronics [4], Elastronics [5], Membrane Switches, 3D Electronics [6], E-Textiles [7], In-Mold Electronics (IME)[8] and Plastic Electronics [9]. The intent of noting these terms is not to sow confusion but rather to highlight the challenge of succinctly defining and categorizing new and emerging technologies.
Advantages of printed electronics
Rigid and flexible PCBs have been in high-volume production for decades. They are a foundational element in almost every electronic device in existence today. There are industry standards, a well-established supply chain and an enormous existing manufacturing infrastructure. So, what’s the pull, promise and potential for printed electronics? The answer depends on the device you’re designing or manufacturing.
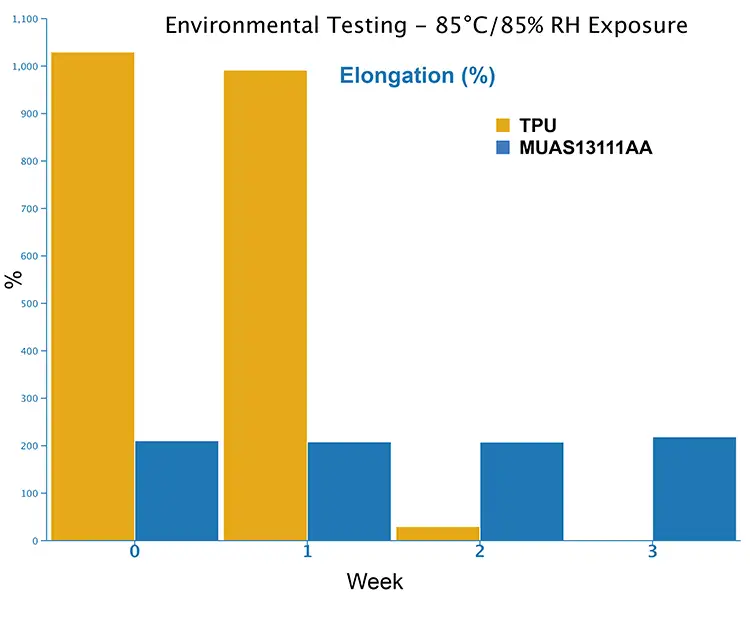
In some cases, it’s the “print-on-demand” feature that’s attractive (particularly with jetting-deposition processes.) One can make functional printed circuits in minutes to hours, rather than the days or weeks typical of traditional PCB manufacturing. Some printing technologies offer the potential for very high-speed / low-cost circuit manufacturing with roll-to-roll (R2R) printing and lamination processes familiar to the converting industry. Without the need for stripping, etching and plating baths, printed electronics can offer environmental benefits over traditional PCB manufacturing. Printed electronics also can enable circuit and device form-factors that simply are not available from today’s PCBs.
Soft circuit materials
The vast majority of PCBs produced worldwide are hard, flat and rectangular. But interestingly, people are not hard, flat and rectangular! Neither are their cars, airplanes, toys, bridges, roads, pets or farm animals. In fact, most of the world is NOT hard, flat and rectangular, and this fact represents one of the tremendous opportunities for printed electronics. There is an enormous unmet need for more conformable, pliant and even stretchable circuits in industries ranging from health and wellness to aerospace, automotive and IoT. Currently, the standard PCB industry can offer flexible circuit boards based on traditional print-and-etch technology. These typically are constructed of polyimide-film substrates with copper foil on one or both sides. While compatible with conventional PCB fabrication, they are constrained by the physical limitations of both the polymer film and the bulk copper.
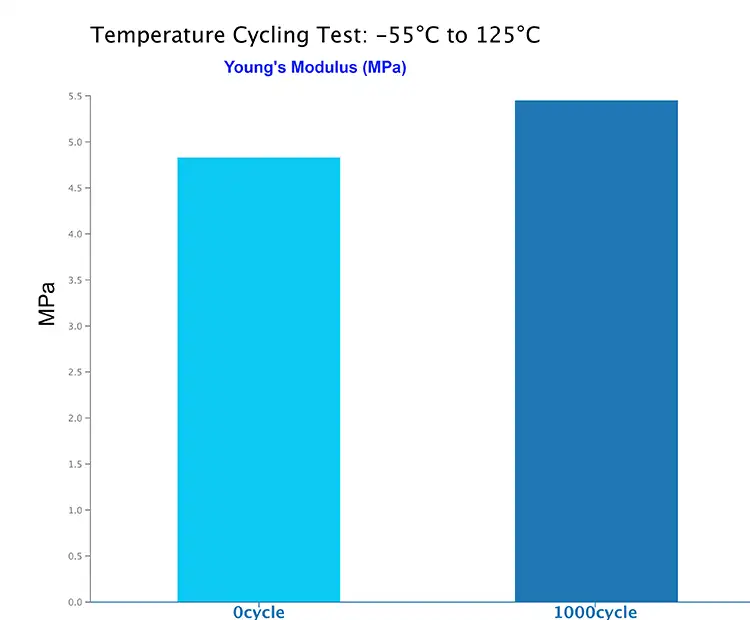
Printed electronics offer an alternative. By combining soft, pliable and even stretchable substrates with soft, pliable and even stretchable conductors, amazing new device form-factors are possible. Pliable circuits are more “organic” in nature; they can accommodate a wide variety of non-planar topography. They can be bent, twisted, folded and even stretched to enable any number of device form-factors.
Most work in the field of soft circuit materials has been based on thermoplastic chemistry. A thermoplastic polymer is a long-chain polymer that softens (melts) above its glass transition temperature and re-solidifies below it, with no change in chemical or mechanical properties. Wax is a good example of a thermoplastic material: it liquifies when it gets warm, hardens into a solid when it cools and can (theoretically) transition between the two states an infinite number of times.
PET is a common thermoplastic substrate for many printed-electronic applications, such as membrane switches. While low-cost and readily available, PET is neither pliable nor elastic, making it, like polyimide, unfit for truly soft circuit applications. The current state-of-the-art processes often employ thermoplastic polyurethane (TPU) as a substrate for stretchable / conformable printed electronics. Like PET, these films readily are available but suffer from low temperature tolerance, which limits component assembly to low-temperature conductive adhesives and greatly restricts the end-use applicability. These polymers also exhibit permanent deformation after strain (hysteresis), which can affect performance and reliability.
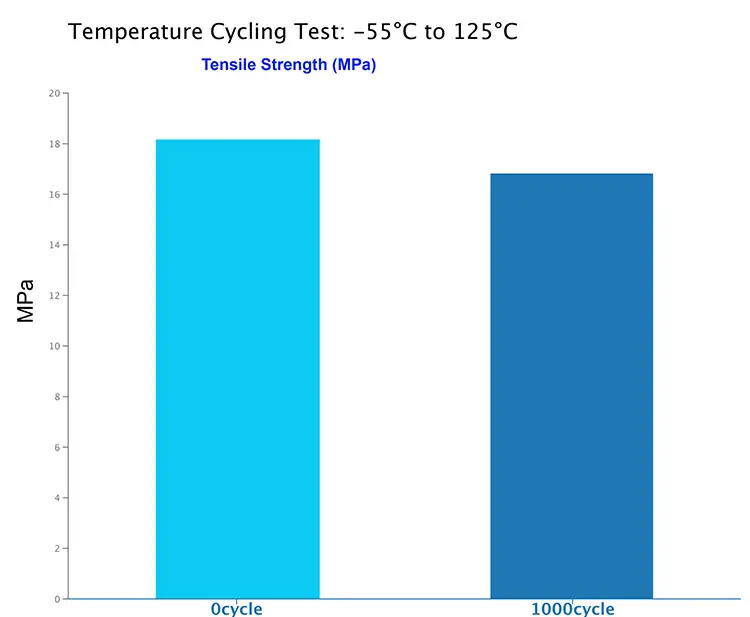
Film based on non-silicone polymer
Researchers at Panasonic Electronic Materials in Osaka (Japan) have developed an entirely new class of soft, stretchable, thermoset films specifically for printed-electronics applications. These materials are based on a novel, proprietary, non-silicone polymer system designed specifically to address the challenges designers and manufacturers face with conventional thermoplastic films. For example, these films have a thermal degradation temperature of greater than 300° C, which enables it to survive many standard electronic-assembly processes including conventional SAC305 reflow temperatures.
Because these polymers are soft and stretchable, they are delivered on a carrier film for mechanical stability. Depending on the end use, the carrier film can be heat-stabilized PET, polyethylene naphthalate (PEN) or polyimide. The circuit fabrication and assembly processes are completed with the carrier film in place. (Typically, the carrier film is removed just prior to final assembly.) By using a carrier film for mechanical stability, the film also is compatible with standard automated converting processes, such as lamination and diecutting.
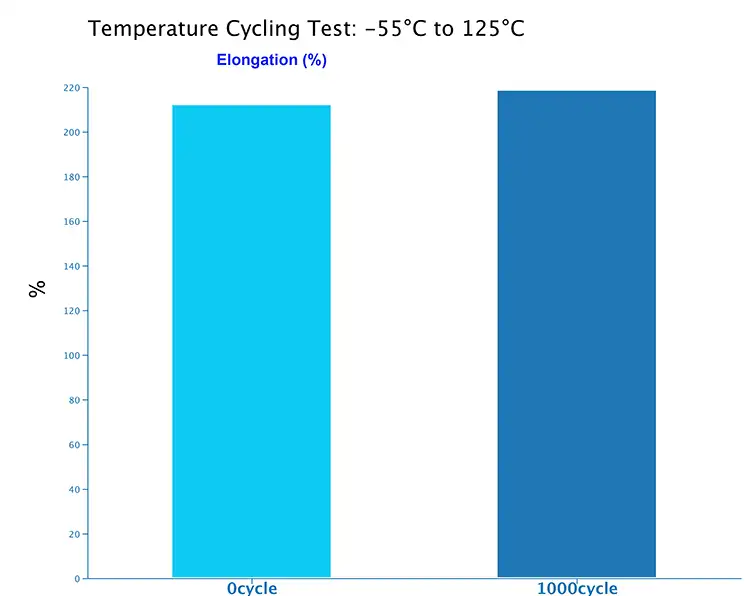
These films exhibit high surface energy, which means they are compatible with many types of functional materials, including printed conductors. Screen-printed silver-polymer composite pastes, liquid metals and jet-dispensed sinterable inks all have been applied successfully. This also means that pressure-sensitive adhesives and other lamination materials bond well without special surface preparation. The films are made on a R2R coater, so they can be provided in either a roll or sheet format as needed by for the manufacturing process; the MUAS13111AA film is 100-microns (4-mils) thick and coated on a PEN carrier.
Evaluation data
Heat & Humidity Resistance: Samples of the film and a representative TPU film (control) were exposed to 85° C / 85% Relative Humidity for three weeks, with measurements being taken at time zero and on a weekly basis thereafter. Figure 1 shows the change in the Young’s Modulus for the two materials over the environmental exposure regimen. By week three, the TPU had degraded to the point where the modulus could not be measured, while the new thermoset film remained unchanged. Figure 2 illustrates a similar trend in tensile-strength measurements. The TPU degrades rapidly while the new film remains unaffected. The elongation-to-break percentage comparison is shown in Figure 3 with similar results.
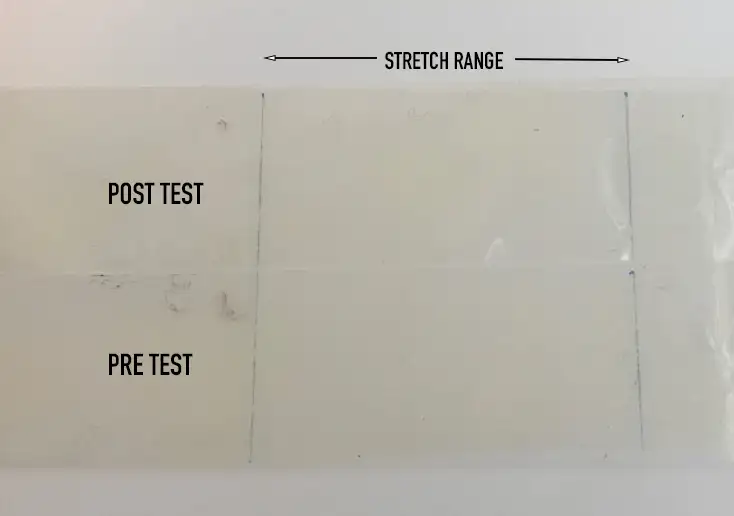
Temperature Cycling Resistance: The new thermoset film was exposed to 1,000 cycles of temperature cycling from -55° C to +125° C. A comparison of initial and final measurements for Young’s Modulus, tensile strength and elongation shows no appreciable change in mechanical performance as shown in Figures 4-6, respectively.
Stretch Cycling: Samples of 48 mm x 25 mm film were subjected to 10,000 stretching cycles (10 cycles per minute). The film length was measured before and after cycling. As shown in Figure 7, the film length before and after stretch cycling is identical, demonstrating that this material experiences negligible deformation (hysteresis) after significant and repeated strain.
Optical Properties: Figure 8 shows that 100-micron-thick samples of the new film have a transparency of approximately 90% across the visible-light spectrum. This film has a 100% polymeric composition with no fillers or particles to diffract light.
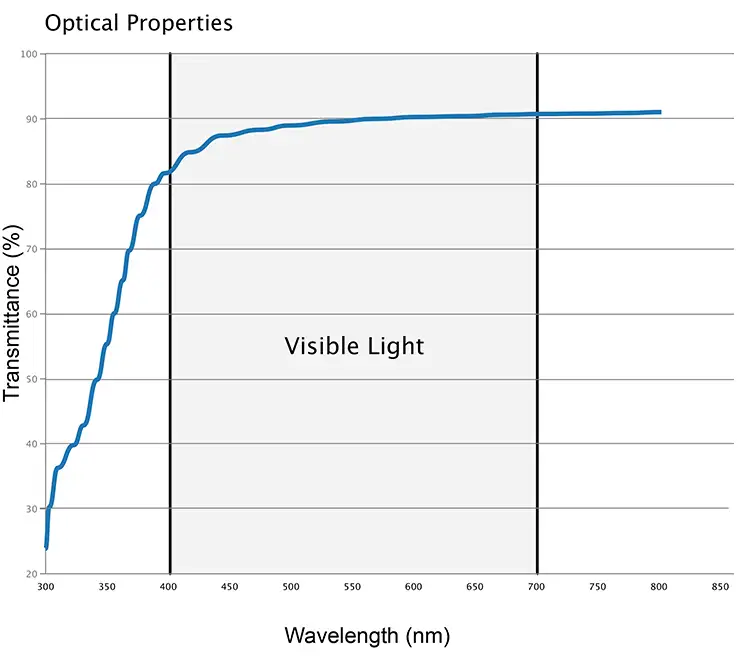
References
- IPC-T-51: Terms and Definitions for Design and Manufacture of Printed Electrons, Published Date 04/01/2022
- NextFlex: www.nextflex.us/about/about-fhe/#:~:text=Flexible%20Hybrid%20Electronics%20%E2%80%93%20devices%20that,stretchable%2C%20conformable%2C%20and%20flexible.
- Stretchable hybrid electronics: combining rigid electronic devices with stretchable interconnects into high-performance on-skin electronics, May 9, 2022, Byeongmoon Lee, Department of Electrical and Computer Engineering, Inter-University Semiconductor Research Center (ISRC), Seoul National University, Seoul, Korea
- Why Flex is betting on stretchtronics for medtech, Medical Design and Outsourcing, September 25, 2017
- Mushroom-like nanowires stand up for elastronics, Materials Today, January 7, 2019
- 3D Printed Electronics, December 2017, Mwamba Bowa, University of Tennessee
- IPC-WP-025: IPC White Paper on A Framework for the Engineering and Design of E-Textiles, Published Date 04/01/2019
- In-Mold Electronics: Short-Term Pain, Long-Term Gain? Plastics Today, November 5, 2021
- Plastic electronics based on semiconducting polymers, First International IEEE Conference on Polymers and Adhesives in Microelectronics and Photonics, 2001, M. Schrodner
Part 2 of this paper will show proof-of-concept parts produced through work with OEMs and contract manufacturers from numerous industries implementing the new thermoset film into product designs.
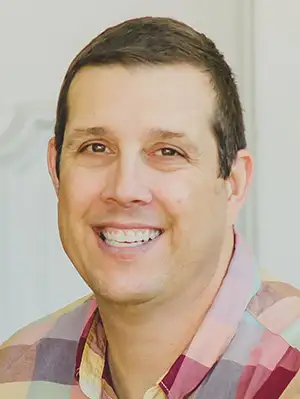
Andy Behr, technology mgr.-Panasonic Electronic Materials Division (Osaka, Japan), leads a research and marketing team based in Silicon Valley that develops leading-edge materials for electronics and other emerging applications. He holds a Bachelor’s degree in Biology and a Master’s degree in Business. Andy has more than 25 years of experience in material technologies such as films, tapes, adhesives, encapsulants and conductive materials. He holds patents and has authored many technical papers. Andy can be reached at email: [email protected] or https://na.industrial.panasonic.com/products/electronic-materials.