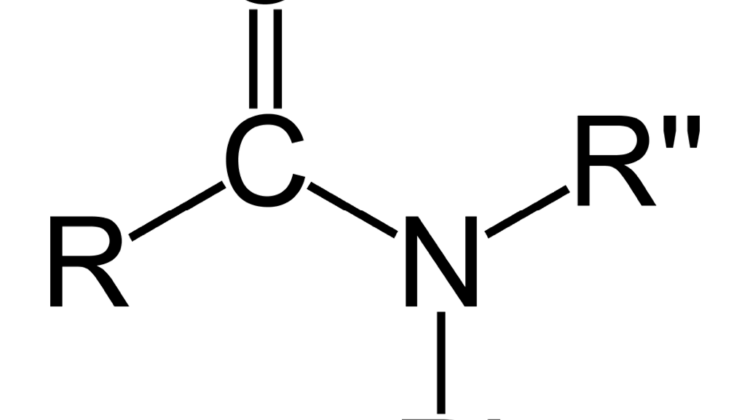
By Kevin McKell, vp-Technical Sales; and Kim Bredgaard, project sales mgr., Vetaphone AS
Introduction
In a world that is becoming more environmentally conscious by the day, there is a moral and commercial obligation on all manufacturers to conform to legislation and public pressure by developing products and processes that are “greener.” In the case of packaging, which as a sector continues to grow globally at an exponential rate, the reduction of solvent use in all aspects of the production process is a pressing issue. The situation is thrown into sharper focus with the development and use of substrates that are more complex in their chemical composition and are required to perform an extended range of packaging requirements that create a critical need for higher surface energy.
There are many reasons for eliminating solvent from the production environment, including safety (removing the risk of an explosion) and added cost (both using it and disposing of it to meet local legislative requirements). Historically, the desired switch from solvent- to water-based technology in inks, lacquers and adhesives has proved problematic, with many of the favored plastic substrates unable to react with oxygen using the well-proven corona surface-treatment process.
For example, corona treatment of a popular material like biaxially oriented polypropylene (BOPP) films will achieve a maximum surface tension value of only 46 dyne/cm – a level too low for its intended further processing. Options are to use a substrate that already is primed or add a primer layer in the production process, but both have disadvantages: the primer often also is solvent-based; it adds thickness to the substrate; and both options add cost to a price-sensitive process. What’s required is a process that allows the use of a cheaper material with no reduction in performance.
The solution to these problems is plasma surface treatment. Plasma is not a new technology in itself, but its latest developments offer a higher level of sustainable surface tension that takes it beyond the capability of corona surface treatment in a growing sector of the packaging market.
By way of example, we will use BOPP as the material in question, owing to its popularity in the packaging sector.
Corona and plasma: The science behind the processes
To fully understand what plasma does, we have to go back to corona treatment where carbonyl groups are created on the surface of the polymer. This happens because O2 is more reactive than N2, even though about 80% of air is N2. But nitrogen is an inert gas, which means that it doesn’t react under normal atmospheric pressure and ambient temperature.
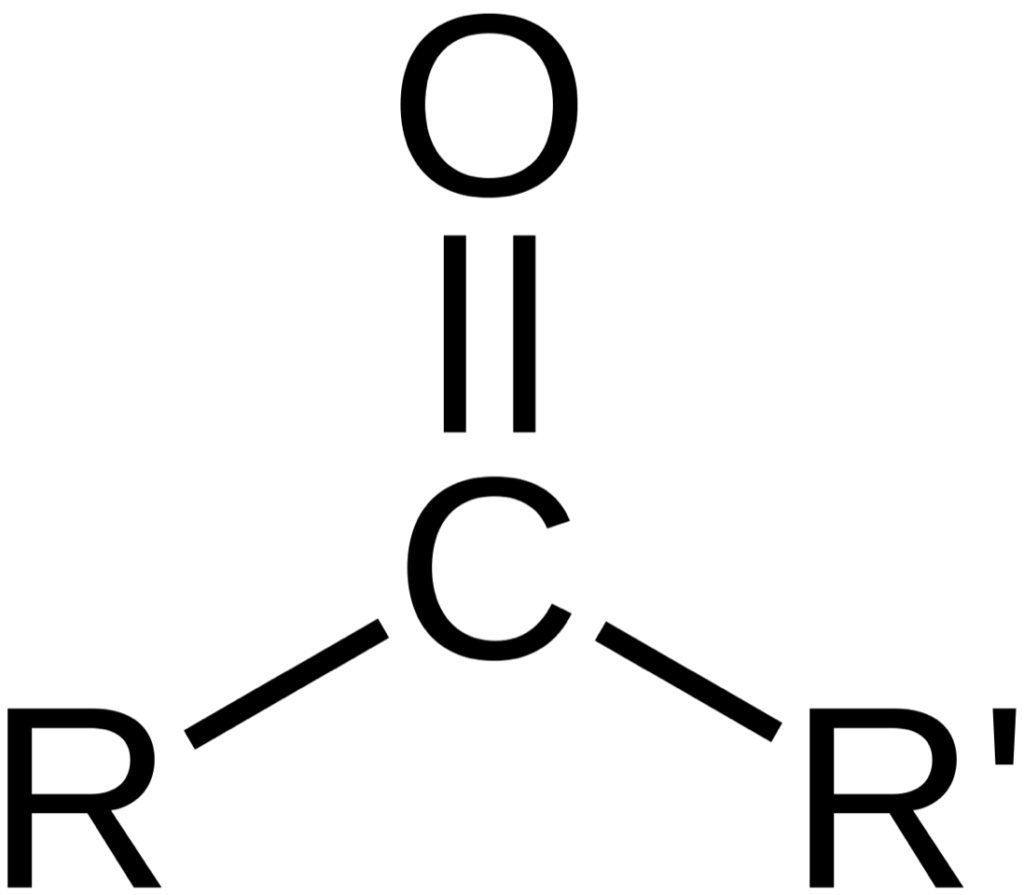
In corona treatment, bombarding electrons onto the surface of the polymer cuts the long macromolecules. This is called a chain-scion-process, and it creates free radicals such as CH3 and CH2, as well as others like CH2-CH2, which interact with the O2. The oxygen also splits into O° and a second O°, and this atomic oxygen is subject to reaction with the free radicals of the polymer, creating carbonyl-groups.
In this example (see Figure 1), R can be a CH3 group from the polymer hydrocarbon-chain or even a CH2-CH2 group. The fact is that the oxygen is not in a stable configuration and will give up its double-bond to become a radical with a free electron. The result is a carbonyl-group that will react with ink, glue or hot-melt adhesive. The side product, ozone, is formed in the same way, by recombining oxygen-radicals (O°) with each other to form O3 – so O° + O° + O° = O3.
In plasma treatment, as much of the oxygen as possible is removed until only a few parts per million (PPM) remain. Now the nitrogen has to play a role because the reactive oxygen has been removed. This creates amines, amides and imides on the surface, thus replacing the carbonyl-groups.
The amines are based on three different types (see Figure 2). There is the primary amine, where the R1 is the link to the polymer (carbon-based CH3-group). The two hydrogen atoms are free in the air and are available to react.
The next is a secondary amine, which has two R-groups fixed to the polymer and only one hydrogen atom free to react (see Figure 3).
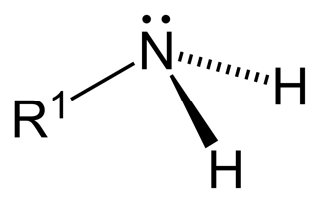
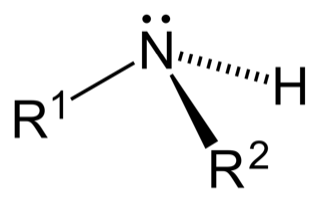
And the next is the tertiary amine with three R-groups fixed to the polymer (see Figure 4). Because of their available functional groups, all three types of amines will react differently with ink, glue or hot-melt adhesive.
In the case of amides, the R-groups are bonded with the polymer (mostly two – that will be R’ and R”). The carbonyl-group only has one oxygen atom, but it is highly reactive to ink, glue or hot-melts (see Figure 5).
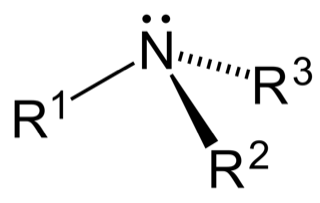
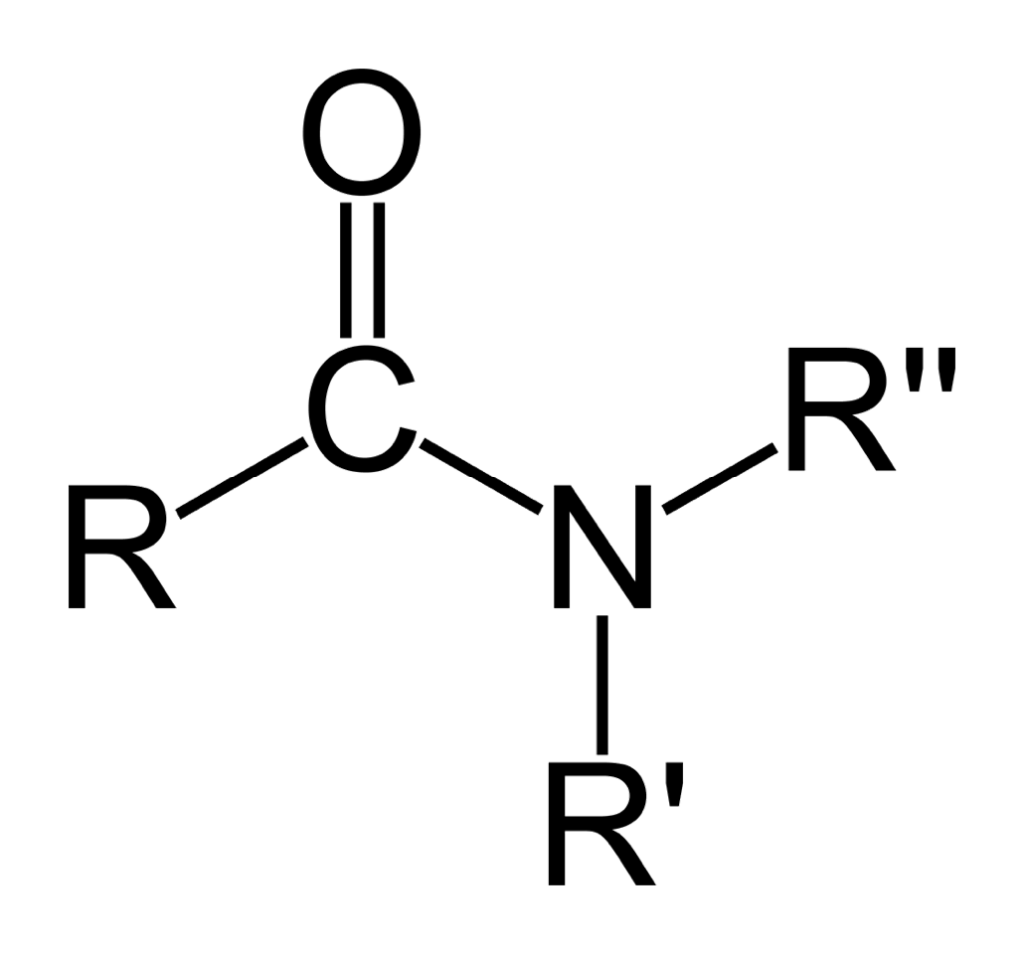
With imides, there are two carbonyl-groups with two reactive oxygen atoms. This configuration makes it the strongest component to react with ink, glue or hot-melts. Because they are highly polar, they easily are soluble in polar media, so when the ink, glue or hot-melt adhesive is polar, this is a good configuration to use in plasma treatment.
It’s all about chemistry and control
There are two key factors that contribute to the success of modern plasma treatment: 1) The need to understand and embrace the chemistry behind the process; and 2) The fine control that allows for a degree of “tuning” the process to achieve the desired result. Merely creating the required molecule groups is not enough on its own. It requires detailed knowledge of how to treat the surface to achieve an even dyne level and use the lowest quantity of consumables if it is to be the best and most cost-efficient product. The secret lies in how to create the correct mix of molecule groups on each specific material in a highly controlled atmosphere.
Surface treatment is not just a function of creating a certain level of surface tension, it also is important that the level is maintained to ensure consistent performance by the substrate in downstream processing. This is called “aging” and should be monitored closely. All plastic substrates that have been surface-treated will age, but not all at the same rate, with those that were corona-treated aging faster than those that were plasma-treated, and from a lower baseline. The aging process relates to the migration of additives in the substrate back to the surface after treatment. With corona treatment, this begins immediately after treatment and, depending on the amount of additive, can be measured in a few hours or many weeks.
The polymer aging process is entirely natural. As soon it is produced in the form of film, sheet, cups, boxes and so on, it starts to degrade slowly due to temperature and pressure changes; humidity also has a great effect on degradation. But most important is UV-light and oxidation of the surface that destroys the upper layer of macromolecules. This process is called natural degradation and ages the polymer. Aging not only changes the properties of the polymer, it will eventually change it into carbon, although that could take many years.
To prevent the natural aging process, polymer manufacturers use a range of versatile additives to overcome the problem.
These include:
- Antioxidants
- UV and light stabilizers
- Heat stabilizers
- Anti-static agents
- Plasticizers
Every polymer contains a certain quantity of additives, depending on its intended application. When film is treated and the radicals are formed on the surface, migration of additives toward the surface begins immediately. The additives are seeking a kind of equilibrium by reacting with the radicals formed during the treatment process. This equilibrium results in the additives blocking the surface of the polymer, while the surface tension simultaneously reverts to its original dyne value. All forms of radicals eventually disappear.
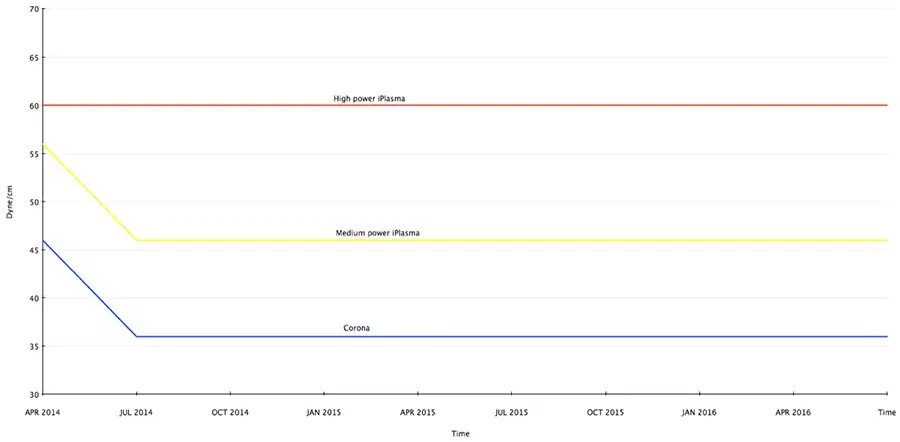
The polymers treated with the plasma process have a higher proportion of nitrogen-related bonding on top of the surface and fewer reactive oxygen groups. This means the outer layer of macromolecules is less subject to penetration by the additives. The more power applied to the polymer film with plasma treatment, the more radicals of nitrogen-related bondings are formed, and the less they are permeable to additives. This means that the aging process is slowed down and can even stop entirely.
All material will age to its native level, which in the case of corona-treated BOPP is around 32 dyne/cm. Figure 6 illustrates the comparative aging of the same material after plasma and high-power plasma treatment. The line graph shows the corona-treated material decaying within two months back to its native 32 dyne/cm. Medium-power plasma also decays at a similar pace, but the aging stops at a higher level at 46 dyne/cm, where it stabilizes. However, with high-power plasma, there is no aging. The 60 dyne/cm achieved on BOPP stays at that level, even after 18 months. For this reason, it is not essential for plasma to be an in-line process.
So, not only can plasma achieve a higher dyne level but also a longer-lasting dyne level. Materials other than BOPP that have so far proven to obtain higher dyne levels with plasma are polypropylene (PP), oriented polypropylene (OPP), polyvinyl chloride (PVC), polyethylene terephthalate (PET) and polyvinylidene chloride (PVDC). And that’s just the beginning, because as more materials with new recipes become available, technology will be developed and adapted to treat them.
The right process at the right time
So, why is plasma surface treatment so successful and becoming a highly valued process in the printed packaging sector? Because it solves the problem of how to replace solvent-based technology with more eco-friendly, water-based inks and lacquers with no drop-off in quality or performance of the end product. There were two fundamental issues: 1) How to achieve and maintain a higher dyne level than corona could offer; and 2) How to cope with a water-based product’s inability to react with oxygen. The fine tuning of gases in a controlled environment that removes the oxygen is the solution, and that process is called “plasma.”
But, as with all surface treatment, the key to success with plasma is rigorous testing in a specialist laboratory. This allows for various gas mixes and power settings to be applied to the chosen material, with adjustments made “on-the-fly” and all results logged for detailed analysis. There is no “one-size fits all” – it is more a highly controllable and variable process that demands a degree of specialist in-house knowledge and expertise. For this reason, it is highly suited to product development because it narrows down the process window to a point where it becomes commercially viable to commit to the investment required for full-scale commercial production.
Early plasma systems often posed as many problems as they solved, with poor control of the atmosphere and high gas consumption leading to an extended ROI. Those days are over. Not only is the gas consumption significantly lower than before, but the atmosphere now is strictly controlled during the entire production process, with all the influential factors closely monitored and the gas flow and mix adjusted accordingly. It is fair to say now that plasma treatment has come of age because its extended-surface treatment capability gives greater scope to plastic material than was previously possible and brings commercial viability to a new range of packaging opportunities.
Kevin McKell, vp-Technical Sales at Vetaphone AS (Kolding, Denmark), is a mechanical engineer by training, with a Bachelor of Science from the University of Strathclyde (Glasgow, Scotland). He has first-hand experience in design and manufacturing. His skills include health and safety, personnel management, team building and his current role in international sales, where he is engaged in pioneering new markets and establishing and building effective sales channels. McKell can be reached at 312-803-3691, [email protected], www.vetaphone.com.
Kim Bredgaard, project sales mgr. at Vetaphone AS, has special responsibility for plasma-treatment technology. Previously a hardware engineer with SAAB Danmark (Infocom) responsible for designing units for secure, tactical voice communication in Operations Rooms for the Navy and Military Air Traffic Control, he moved on to become product manager, defining the architecture, technology and feature set of the latest generation of these systems. Bredgaard is a qualified electronic tekniker from EUC Syd in Denmark. His role at Vetaphone is to act as the interface between customer and R&D to interpret requirements into product specification and generate product roadmaps. Bredgaard can be reached at 312-803-3691, [email protected].