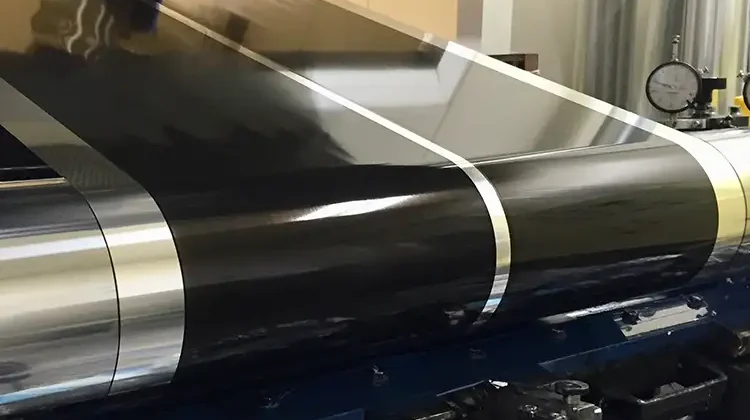
Edited by Editor-in-Chief Mark A. Spaulding
Editor’s Note: This Q&A report presents the views of Anthony Sudano, a mechanical engineer and business consultant with more than 30 years of experience in the converting, packaging, medical and battery industries. Since 2007, his consulting firm has helped advanced-technology battery- and ultracapacitor-manufacturing companies – from startups to multi-nationals – with business plans, funding applications and optimizing manufacturing processes.
Converting Quarterly: Please provide a 30,000-ft overview of the global battery field when it comes to types, end-use markets, new applications and regional demand.
Sudano: In the rechargeable battery space, the battery technology has moved rapidly from Nickel-Metal Hydride to Lithium-Ion (Li-ion) technology. Other technologies, such as Lead Acid, still are prominent for applications such as car-starter batteries, lift trucks, stationary backup power, etc., due to its still lower cost and excellent low-temperature cranking characteristics. However, Li-ion technology has seen significant cost reduction over the past years, which make its high energy density and good cycle life very compelling arguments for integrating this into existing and new applications, such as electric vehicles, stationary energy-storage systems and consumer products.
The growth of renewable energies – namely solar and wind power, which generate electricity periodically – marry well with batteries, which act as energy-storage buffers, thereby leveling the difference between the offset periods where energy is generated, either during daylight hours or when the wind blows, respectively, to periods of electricity demand.
Batteries of all shapes and sizes for the numerous applications are in worldwide high demand. Historically, Japan, South Korea and now more specifically China have been producing the majority of battery cells, which can be integrated into larger battery systems, depending on the type of application. However, over the past few years, battery-cell factories have been, and continue to be, built closer to points of use, such as Europe and the United States, with other continents or countries starting to build their own battery-production capacity primarily to overcome transportation costs or country-imposed import tariffs. Such areas include India, Brazil, Australia, Eastern Europe and others.
CQ: What are some of the key trends for battery manufacturing in general (solution and vacuum coating) and R2R processing specifically?
Sudano: Li-ion battery fabrication starts with the application of mixed slurries onto thin foils. Thin Aluminum and Copper foils on the order of 6-12 microns thick are used for anode and cathode electrodes, respectively. The mixed slurries are solution coated via slot-die first onto one side of the foil web and forced-air dried in long drying tunnels. The back side then also needs to be solution-coated and dried in the same manner. Because both sides of the foil need to be coated, this can be done either in two separate passes through the same machine or through two successive coating and drying stations. This is done to reduce roll handling and increase productivity. Running speeds are a function of the amount of slurry applied to the foil substrate, which is a function of power and energy characteristics required by the application, as well as the length of the drying tunnel. The longer the dryer, the higher the operating speed for a given amount of slurry applied. This method is the baseline or incumbent R2R technique for producing battery electrodes.
A slight improvement to the described process is to simultaneously apply slurry to both sides of the foil and dry the double-side coated web through one flotation dryer. The method reduces the manufacturing & capital-expenditure cost as well as required building space by eliminating one of the two drying tunnels per machine.
As alternate to the above described solution-coating method is a dry-powder compression and lamination to the foil substrate. This method is dependent on the materials used – in particular, the binding agents and mixing preparation. The dry materials need to be able to bond and hold together under compressive force. Fibrillation of the binding material helps anchor the other dry powders. Feeding the dry mixture through a set of compression rolls forms a thin film of the active battery electrode. This film then is laminated onto the foil substrate. As both sides of the foil substrate need to have the active electrode, this electrode lamination also can be applied to both sides of the foil simultaneously. Processing the active materials in this dry manner eliminates the need and use of solvents, which are costly and also need to be treated either by incineration or by condensation and recovery, not to mention the avoidance of batch mixers and long costly dryers.
This dry-processing method first was developed by Maxwell Technologies, which was acquired by Tesla in 2019, in large part due to this reason.
Vacuum coating also is used in specialty-battery products, such as small surface-mount energy devices in the electronics field. With this method, specialty coatings can be applied to current collector films to decrease internal resistance and fabrication of solid-state batteries. Unlike vacuum deposition of metallized films for the packaging or vapor-barrier materials industry, the amount of material needed to be deposited for battery applications is rather high, which leads to lower operating speeds and higher processing costs, especially when compared to the baseline, solution-coating method. This is the main reason for this method being relegated to specialty – albeit high-value – devices for now.
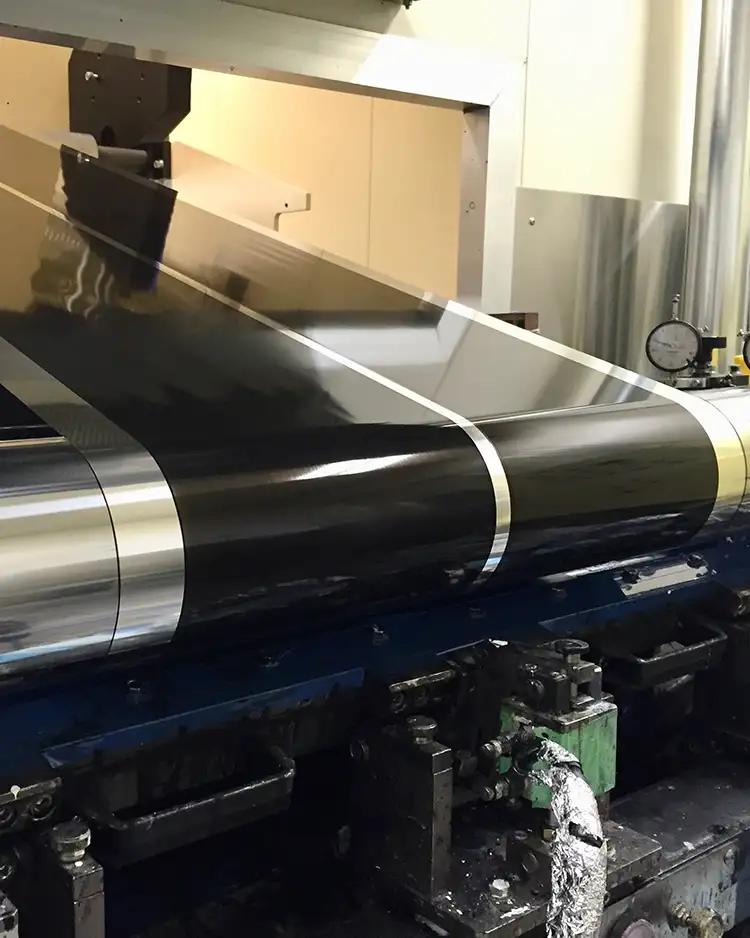
CQ: What are some upcoming battery-technology developments that could displace incumbent technologies?
Sudano: While still being part of the Li-ion family of chemistries, one variant with significant potential is the Lithium-Silicon battery. In this configuration, the graphite anode is replaced by a silicon anode. Silicon has approximately 10 times the specific capacity of graphite, which leads to much greater potential for high energy density. Its drawback is that during battery charging, the silicon will expand to 400% of its original dimensions. This leads to increased internal pressure and/or swelling of the battery. Microscopically, the expanding silicon, which is mixed with other conductive and binding agents, will break apart from its constituents, thereby interrupting the conductive network within the electrode and leading to higher internal resistance and heat generation. Together, this results in premature battery failure, which clearly is counterproductive.
Incremental improvements are being achieved by mixing small quantities of silicon within the baseline graphite anode on the order of 5 to 10% silicon content. Although still far from reaping the maximum benefits of silicon, this improves the cell capacity slightly while minimizing the undesired effects of swelling and micro-cracking within the anode electrode. Work persists to develop ways for the silicon particles to be shaped, such as by using silicon nanotubes, which absorb the swelling internally, thereby avoiding the risk that the outside of the silicon nanotube will break away for its neighboring particles. The replacement or addition of silicon within the anode electrode is accomplished using essentially the same slurry-mixing and electrode-coating R2R equipment, as well as the same cell-assembly processes.
Yet another technology that is garnering much attention is a Lithium metal/solid-state electrolyte battery. In this technology, liquid electrolyte is replaced by a solid electrolyte, which can be a polymeric or a ceramic. The main advantages with this technology compared to the incumbent Li-ion technology is that the organic-solvent-based electrolyte is replaced by a non-flammable solid, which is safer and more energy-dense. Because no liquid needs to get into the pores of the materials, a metallic-lithium anode can be used, offering a larger quantity of lithium in a much thinner amount of material. As Lithium metal is extremely light and also a great electronic conductor, the overall cell dimensions become smaller and lighter for the same amount of energy. Conversely, more energy can be extracted for a lighter cell of the same size.
CQ: Is energy efficiency, material downgauging and/or sustainability impacting R2R battery manufacturing and how?
Sudano: A good example of downgauging of materials is in the use of thinner-gauge aluminum and copper foils as current collectors. Their main purpose is to act as the electrical conductor for a battery cell. In many applications, they are oversized, but have historically been used due to their market availability and their R2R web-handling robustness. Finding high-quality, pinhole-free, thin foils was not easy. Also, handling very thin foils in R2R processes demands very high-precision equipment with excellent and costly tension-control systems. However, as demand grows, quality foils – either rolled or electro-deposited copper foils in the 6- to 8-micron range – have improved in quality even further and now are more readily available. By the same token, R2R-coating machines have been built to be able to handle such foils.
CQ: What new machinery and material developments are there now – or may be coming – for flexible, thin-film batteries?
Sudano: Unlike Li-ion batteries, which use a graphite-electrode on a copper current collector, solid-state Lithium batteries use a metallic-lithium anode. The non-rechargeable type uses a rather thick Lithium-metal foil, which is relatively simple to produce using cold-extrusion pressing. For rechargeable batteries, where high energy density is of greater importance, there is an advantage in using thinner amounts of lithium. Rolling or laminating ultra-thin Lithium foil <20-30 microns is rather challenging. An alternate approach is to vacuum-deposit Lithium onto a thin copper current collector. In many cases, <2-10 microns of lithium on either side of a copper foil is all that is required for such batteries. This vacuum-deposition process specifically for the Lithium-metal anode can become quite a coveted process as the tendency toward lithium-metal, solid-state batteries continues to progress.
CQ: What new successes have there been, and what is the outlook for batteries for hybrid and all-electric vehicles to overcome possible future shortages?
Sudano: As with most new technologies, time and competition spur technological advancements and lead to lower cost. My favorite example of this is the plasma television. Early adopters paid exorbitant amounts of money for the first flat-screen plasma TVs. Nowadays, you can’t even find a picture-tube television, and LCD TVs can be had for a song. A similar downward-cost trend is being experienced with Li-ion batteries. In 2010, they sold for ~$1,200/kWh (per Bloomberg New Energy Finance). Nowadays, it’s down on the order of $150/kWh and projected to be <$75/kWh by 2025. Keep in mind that an electric vehicle can have a 50- to 100-kWh battery pack on board, depending on the size and model.
What has led to this reduction in battery pricing is the overall improvement in many of the materials’ performances, mass-production and cost reduction in materials and components. Large manufacturing facilities are highly automated, so labor cost has drastically declined in significance, as compared to years ago. Of the overall cost of a battery, about 80-85% is the materials, 5-10% labor, and the rest being energy, capital-depreciation expense and overhead.
With materials playing such a key role in the overall battery cost, the introduction and increase of recycled-materials content within batteries will help reduce costs as the industry grows.
CQ: What solutions are being worked on for recycling of any and all kinds of batteries?
Sudano: Whenever there is a market demand, solutions generally get developed. Recycling in the Lead-Acid battery industry is quite prominent, with a high percentage of materials being recycled and re-used. The Li-ion industry has not produced as much volume of batteries yet; however, that is changing exponentially due to the recent rise in large electric-vehicle batteries hitting the market. As these batteries start coming to their natural end of life in the years ahead, there not only will be a need to recuperate and slow the flow of such batteries from hitting landfills, it also will be vital to recover the valuable materials and recycle them into new batteries – an extreme benefit commercially.
The opportunity for recuperating valuable materials, not to mention the environmental factors, is seen as a major business. In fact, making batteries will require large amounts of rare earth materials, whose supply may eventually diminish. Recycling batteries will serve as a double advantage where recycled materials can be used in the fabrication of new batteries. Currently, about 5% of active materials used in new-battery fabrication comes from recycled materials. The reason? There simply aren’t that many batteries yet available to recycle. However, as the industry grows and more batteries start reaching their end of life, there will be more expended batteries to recycle which, in turn, will produce more available recycled materials to be made into new batteries. At some point, the majority of materials used to make new batteries will be from recycled sources – not unlike new paper and paperboard products being made with 100% recycled content.
Pyrometallurgy (the use of high temperatures to extract and purify metals) is employed to dissociate certain elements of a battery. This is effective; however, there is a limit to which and how much of the metallic components (such as Nickel, Cobalt, graphite, etc.) within the battery electrodes can be recovered. New methods using hydrometallurgy are increasing the amount of materials being recycled to >90% as well as using low-emissions processes. The result is that as needs and opportunities are identified, such as with recycling of battery materials, scientists and companies will work on developing new and more-effective methods to improve the default means, thereby leading to cost and environmental benefits.
Anthony Sudano, principal of Sudano Consulting, Inc. (Montreal, QC, Canada), is a mechanical engineer (Concordia University – Montreal) and business executive with over 30 years of experience in automated machine design & fabrication for the converting, packaging, medical and battery industries. He worked at a startup company, developing new manufacturing processes for a rechargeable Lithium-metal, solid-state, electrolyte battery technology en route to building the world’s first rechargeable SSB commercial manufacturing facility. Since 2007, Sudano has run a consulting firm helping advanced-technology battery- and ultracapacitor-manufacturing companies with business plans, funding applications, manufacturing audits, and developing or optimizing manufacturing processes. He also is a co-inventor on several patents and a contributing author to Linden’s Handbook of Batteries: 5th ed. on the subject of battery manufacturing. as well as a speaker at various industry conferences. Sudano can be reached at +1-514-235-4646, email: [email protected], www.SudanoConsulting.com.