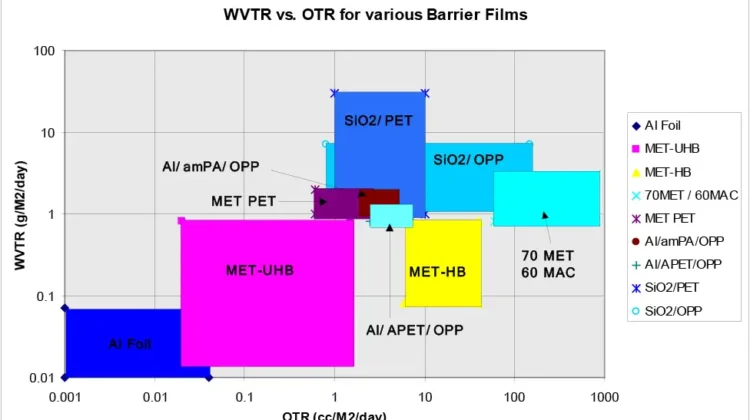
By Eldridge M. Mount, Ph.D., president, EMMOUNT Technologies
This paper will review the development history, observations and discoveries of an industrial research and development group that demonstrated the source of metallized-film gas-barrier properties. These discoveries lead to a range of patents and commercial, metallized packaging films. The information still is of value to film manufacturers, converters and material end-users in determining the most appropriate product design and converting practices to optimize barrier packaging.
Introduction
Metallized-polymer films supply an electric or static barrier due to conduction of the metal layer. In capacitors, the metallized film acts as an electron storage device, perhaps the first use of metallized films to replace metal foils.
Metallized films in packaging are in broad use today due to their wide and varied barrier profile. Of all the metallized-film barriers, one of the most important is the barrier to light. Either to hide what is in the package or to protect the products from damaging wavelengths of light. Without this light barrier, the shelf life of most products would not be sufficient to see the benefits of the additional gas-barrier properties. Light-sensitive foods and products have long been packaged in opaque containers, such as metal cans, or light-filtering packaging, such as tinted glass bottles.
But for most packaging applications, after light, we are interested in the gas barriers of metallized films. The principal gas barriers of interest in packaging are oxygen and moisture (water vapor), followed by chemical (flavor and aroma) barrier. Figure 1 is a composite graph of metallized films’ moisture barrier against oxygen barrier first published in 1996-67 [1] and updated over time. Figure 1 clearly shows that the moisture and oxygen barriers of many different polymer films vary over wide ranges. Clearly, the presence of the aluminum layer may not be the controlling factor in metallized-film barriers. Thus, the question, “What is the controlling variable in metallized-film barriers?” I believe I can answer this question.
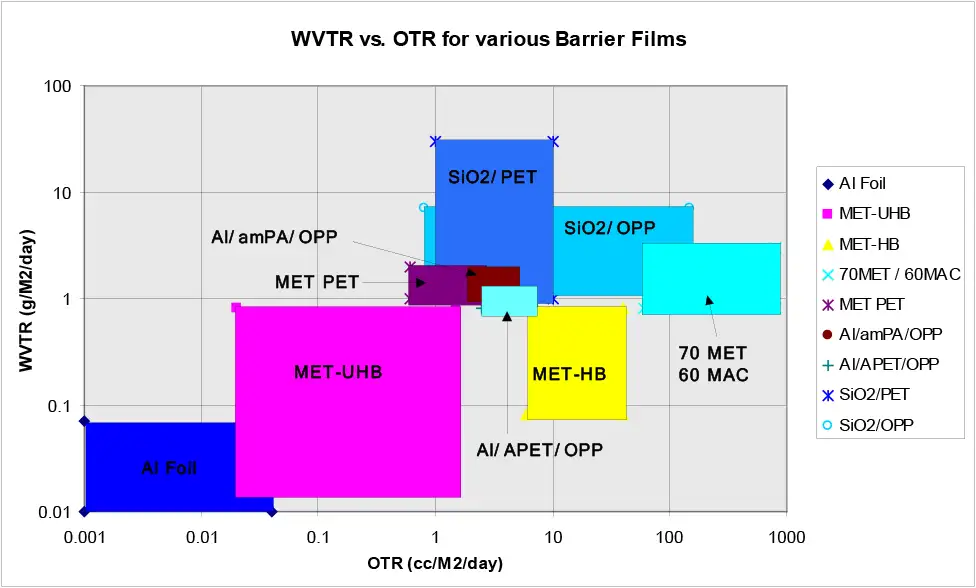
Concerted, cooperative development
What follows is a bit of the history of metallized-film development that I participated in to highlight the observations and discoveries my coworkers and I made of the source of metallized films’ barrier properties. This was a multi-year, multi-person, multi-hypothesis and even a multi-competitor effort. Most of these discoveries are well referenced in the patent literature of metallized films. Interpretation of the observations on film barriers generally are my own.
When I began my development work at Mobil Films on metallized packaging films around 1984, there were several metallized films in commercial existence: metallized-PET (mPET) and a few emerging, potential metallized-OPP (mOPP) products [2,3,4]. We were searching for a 3-layer coextruded-film product (see Figure 2) which was competitive to mPET. The product would give a heat-sealable, metallized, lamination film with metal-fracture resistance in laminations and excellent metal adhesion (see sidebar on metal fracture). It was assumed that the gas barrier properties would equal that of mPET, i.e. the presence of the metal layer supplied the barrier properties. At this time, we already had experienced a fitness-for-make failure with a film using a corona-treated, metal-fracture-resistant, homopolymer metallizing skin. This failure was due to surface scratching of the homopolymer skin in the machine direction orienter (MDO) and backside treatment giving metal pickoff in the metallizer – neither of which could be effectively eliminated in production. Both defects also caused poor appearance and gas-barrier results.

Simultaneously, our Canadian plant was beginning to produce a metallizing base film with a corona-treated copolymer metallizing surface. The use of a copolymer metallizing skin eliminated the MDO surface defects, which would be “erased” as the copolymer skin would melt in the oven of the transverse direction orienter (TDO). However, the film still suffered from backside corona treatment and metal pickoff clouding the barrier results. However, the backside treatment could be minimized, and the metal fracture could be controlled by careful tension control on extrusion laminators.
So, a promising product design?
To eliminate the catastrophic barrier results of backside corona treatment, I had flame treaters installed in manufacturing and suddenly there was the potential for a fit-for-make and fit-for-use product design. As we will see, this also paid unexpected benefits in commercializing high-barrier, metallized-OPP (mOPP) films. The stage was set, manufacturing experiments were conducted, metallized product was produced and statistical process control (SPC) property data was collected and control-charted.
It then was discovered, while the mOPP product’s moisture barrier (WVTR) matched the mPET WVTR data, the mOPP’s oxygen barrier (TO2) was several orders of magnitude higher than that of the mPET TO2 barrier (see Figure 1). This result was not well received, but the SPC data made it irrefutable. See my many Substrates Secrets columns for a long-term emphasis on the importance of control-charting and statistical SPC data (Converting Quarterly 2022 Q1-Q4).
My hypothesis (defense?) was that the TO2 barrier of mOPP was different than that of mPET because copolymer PP was not PET, i.e. the polymer that was the metallizing layer had a role in determining the ultimate gas-barrier profile, not simply the presence of the metal layer. To test my hypothesis (aka “prove my innocence?”), I modified our pilot line to produce the 3-layer structure shown in Figure 3. Two films were produced. One with an amorphous-PET metallizing skin [5] and one with an amorphous-Nylon skin [6]. Both OPP coextrusions gave metallized-film barrier results comparable to mPET (see Figure 1).

A flame-treatment solution
A coworker, Robert Migliorini, hypothesized that the free volume of the metallizing polymer was the source of the better mPET oxygen barrier. He used the new coex-product design to coextrude a metallizing skin of 44% ethylene EVOH [7]. As shown in Figure 1, this EVOH/OPP film produced a metallized barrier comparable to foil and better than all previously tested metallized-barrier films. This discovery highlighted the significance of metallizing surface-polymer chemistry to the final WVTR and TO2 barrier properties. Back then, this discovery was not considered commercially viable due to potential manufacturing costs.
However, consider that EVOH simply is a hydroxylated polyethylene (contains alcohol, -OH, groups) and combine that with the knowledge of my coworkers’ treatment studies. Specifically, flame treatment results in a higher surface concentration of hydroxyl groups than corona treatment’s carboxylic acid end chains. You suddenly arrive at the invention of flame-treated HDPE metallizing skin [8]. Flame-treated HDPE, metallized-OPP films produce material with the moisture barrier of a metallized-EVOH skin and an oxygen barrier between those of mOPP and mPET (see Figure 1) but with a perfect barrier profile for many snack-food packaging applications, extending shelf life and economical to manufacture.
At this stage, it seems that both hypotheses for the control of the barrier properties is not quite settled, and indeed they may actually both be a bit true. Still further, we will see that they work in conjunction with each other, especially for the chemical (flavor and aroma) barrier of metallized films. However, another observation during the metallization process helps to show the significance of surface chemistry over free volume for WVTR and TO2 and adds in the impact of the actual metal layer.
Composite rolls aid experiment analysis
In most of my product metallization studies, I used composite rolls, with each roll containing several separate product designs spliced together into a single roll for metallization. This has the benefit of economy in sample size and metallization sample costs. But the composite roll has the principal benefit of operating at identical metallization vacuum conditions so differences in product design are more easily determined. This is important [9]. My interpretation, from my metallization vacuum-pressure-designed experiment, was that the lower the vacuum pressure during deposition, the “better” aluminum layer that is produced.
To explore the barrier variations between EVOH and flame-treated HDPE films, a composite roll was produced with:
- A threading-leader film to stabilize metallization conditions
- A sample of commodity metallizing base sheet (copolymer metallizing surface)
- A sample of flame-treated HDPE metallizing base sheet
- A sample of EVOH-skin metallizing base film, and
- A final threading leader to allow ending of metallization.
The metallization was started, and an optical density (OD) of 2.0 to 2.2 was established and stabilized on the copolymer base sheet. As the splice to the HDPE skin sample passed, the deposited OD of the film increased significantly, with no evaporation rate adjustments. Next, as the splice to the EVOH skin passed, the OD of the deposit increased again. The OD then dropped back to 2.0 to 2.2 as the final leader signaled the end of the EVOH sample. This indicated that the evaporation rate remained constant during the experiment.
So, at a uniform vacuum pressure and a constant evaporation rate, the two high-barrier base sheets produced higher OD deposits at uniform deposition rates than the commodity base sheet. In short, the aluminum layers deposited were optically denser and, therefore, have a higher refractive index. A higher refractive index implies that the density of the aluminum layer is higher. In short, the metal layers on the flame-treated HDPE and EVOH are denser or “better” for resisting the diffusion of gases.
Impact of surface chemistry on quality
Clearly, the surface chemistry of the metallization surface is controlling the “quality” of the aluminum layer deposited on the film surface and, therefore, the surface chemistry of a metallization surface (polymer type) is the source of metallized-film barriers by improving the metal layer’s barrier. In Figure 1, we find a wide range of metallization surface polymers giving a wide range of oxygen and moisture barriers, but better than most, by a wide margin, are the hydroxylated-HDPE surfaces, EVOH and flame-treated HDPE.
But Bob Migliorini’s hypothesis of lower free-volume effects may play a role with the further improvement of TO2 for an EVOH layer when compared to the flame-treated HDPE films, but not for moisture barrier. Along the same lines, it has been demonstrated that the existence of an aluminum layer on a film does not guarantee there will be a flavor and aroma (chemical) barrier [10]. Here, the chemical resistance of the base film and metallizing skin polymer plays a controlling role – along the lines of solvent resistance of films.
Solvent chemicals for any particular polymer will cause swelling of the base polymer and swelling of the metallizing skins, which will disrupt the integrity of the metal layer at the polymer/metal interface. This is highlighted by the chemical barrier of the metallized-EVOH skin samples [1] when compared to the HDPE and copolymer OPP films. In this instance, the chemical resistance of EVOH will prevent the swelling adjacent to the metal layer and, therefore, prevent the disruption of the metal layer.
Conclusion
It is indeed the presence of the aluminum deposit on the film surface that produces the light, moisture and oxygen barrier of a metallized film. However, it is the surface chemistry of the polymer surface on which the metal is deposited that controls the “quality” of the aluminum deposition and its gas and moisture barrier. I believe that through the studies conducted with my coworkers at Mobil Films, as detailed above and in the patent literature, it is the presence of a hydroxylated-polyethylene skin, such as flame-treated HDPE or EVOH, which produces the highest-quality aluminum deposit in metallized packaging films.
References
- Mount III, E. M., “Aroma and Moisture Barrier Properties of Plain, Coated and Metallized OPP Films,” Technical Proceedings SME Barrier Technology for The Food Packaging Industry, June 4-5, 1997.
- Reid, D.E., US Patent 4,604,322, “Metallizable Polypropylene Film,” Hercules Inc., August 5, 1986.
- All, F., Duncan, G., US Patent 4,345,005, “Oriented polypropylene film substrate and method of manufacture,” Mobil Oil Corp., June 28, 1979.
- Koebisu , et al., US Patent 4,522,887, “Laminated film of biaxially oriented polypropylene and an olefin polymer film,” Toray Industries, Inc., March 19, 1984.
- Mount, E.M., Benedict, A.J.; European Patent 444340; “Metallisable Heat-Sealable, Oriented Polypropylene Film – Has Layer of Co-polyester To Improve Bonding To Metal,” (1991).
- Migliorini, R.A., Mount III, E.M., US Patent 5,591,520, “Multilayer film with metallized layer,” Mobil Oil Corp., October 25, 1991. Migliorini, R.A., Mount III, E.M., US Patent 5,1943,318, “High Barrier Film,” Mobil Oil Corp., April 28, 1995.
- Migliorini, R.A., |US Patent 5,153,074, “Metallized Film Combination,” Mobil Oil Corp., October 6, 1992, EVOH patent.
- Migliorini, R.A., Mount III, E.M., US Patent 5,1943,318, “High Barrier Film,” Mobil Oil Corp., April 28, 1995.
- In an earlier metallization process study conducted about 1986 with 16 BOPP rolls (a Box-Benkin experiment) at Twin Pak with Bob Szoke. The process experiment found that metal barrier was significantly impacted by metallization chamber vacuum level, the lower the better. Bob repeated the experiment with PET film and reported his results at the first Bakish Conference; Szoke, R.L., “Vacuum Metallizing Plastic Films And Papers With Aluminum Control Parameters And Limitations A Converters Perspective,” Proceedings Of the First International Conference on Vacuum Web Coating, Nov. 29 to Dec. 1, 1987, ed. R. Bakish, pp. 149-158.
- Mount III, E. M., Wagner, Jr. J.R., US Patent 5,981,079, “Enhanced Barrier vacuum metallized films,” Mobil Oil Corp., November 9, 1999.
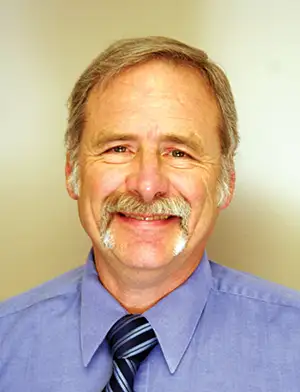
Eldridge M. Mount III, president of EMMOUNT Technologies LLC (Canandaigua, NY), has 40+ years of industrial experience in the extrusion and orientation of polypropylene and polyester films at ExxonMobil Chemical and ICI Americas Film Divs. He holds a Ph.D. in Chemical Engineering from Rensselear Polytechnic Institute. Eldridge writes the “Substrate Secrets” Q&A column and moderates the “Substrates” Technical Topics online channel for this publication. He can be reached at 585-223-3996, fax: 585-919-6504, email: emmount@earthlink.net, www.emmount-technologies.com.