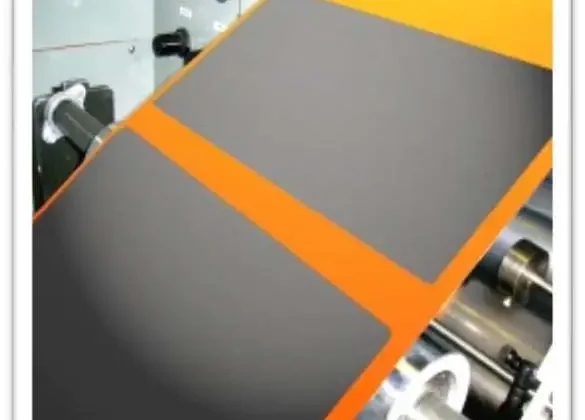
By Sheila Hamilton, technical dir.-Teknek, Inc.
Editor’s Note: This technical paper is based on a presentation at the AIMCAL R2R Conference Asia 2019, held in Daejeon, South Korea, organized in cooperation with the Korea Research Institute of Chemical Technology (KRICT).
Abstract
Contamination, both ionic and particulate, is a major issue in the roll-to-roll (R2R) manufacture of Lithium-ion (Li-ion) batteries. Research has shown there are many opportunities within the manufacturing process itself and within the supply chain for contamination to be introduced into the process. This paper will outline the areas within the process that are sensitive to particulate contamination, highlighting the defects that contamination can cause, with special focus on safety-critical defects. The paper will conclude by discussing the steps that can be taken to reduce contamination throughout the process, resulting in higher yields.
Contamination issues
With the growing demand for Lithium-ion (Li-ion) batteries and the relative scarcity of the materials used in their production, it is vital to obtain maximum yield in the battery-manufacturing process. As in many roll-to-roll (R2R) processes, contamination is a major cause of defects throughout battery production. Contamination results in two major issues – namely, short circuits between the electrodes and poor performance. Both issues result in latent defects that do not become apparent until the product is in use. Short-circuit defects have significant safety implications. Recent incidences of battery fires have resulted in many airlines restricting the carriage of batteries. Latent defects affecting performance, such as dramatically shortened life or poor charge capacity, engender significant loss of customer confidence.
Internal short circuits normally are caused by metallic particles that can penetrate through the separator layer. The resulting short circuit becomes a hot spot within the battery and can initiate a fire through the ignition of solvent-based electrolyte. In 2006, Sony had to recall 10 million batteries after particles of nickel, copper and iron were found in the electrolyte of batteries that had caught fire. There are a very large number of process steps where metal contamination can be incorporated into the battery, one being the slitting/rewinding of the foils which form the collector of the electrodes.
In battery performance, high charge capacity and long life are the parameters which provide a competitive edge, and anything that negatively impacts those parameters can have a significant commercial impact. For optimum performance, the charge transfer between the electrodes and through the electrolyte has to be completely uniform over the whole cell area. Any particle of contamination which blocks the pores in the separator film, through which the ions transfer, causes a distortion in the charge transfer field. Contamination, either conductive or non-conductive, on the electrode surface itself causes a local disparity in the charge transfer mechanism due to dewetting of the electrolyte. Variation in the charge transfer mechanism causes reduction in both charge capacity and in the number of charge/discharge cycles the battery can sustain, thus resulting in a shortened battery life.
Battery structure
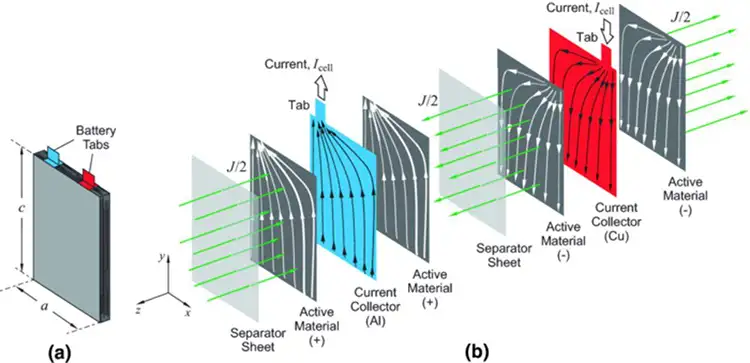
While Li-ion batteries are available in a variety of packages from button cells through pouch cells for high-end electronics to car batteries, they all share the same fundamental structure as shown in Figure 1.
They all comprise an anode and a cathode, which must be kept a fixed distance apart and an electrolyte to transfer the charge from one electrode to the other and back again. In this paper, the focus is on the thin, flexible pouch batteries used in high-volume electronics as the structure of these lends itself particularly well to R2R manufacturing.
In pouch batteries, there are two main components in each electrode – namely the current collector foil, which is used to collect all the charges and transfer them into or out of the battery. Then there is the active material layer which provides the chemical functionality to enable the battery to function. For the cathode, a porous coating of a metal oxide is coated on either copper or aluminum foil, which is between 8 and 18 microns thick. The anode is usually a 10-micron copper foil coated with a carbon-based active material. The base collector foils often are treated to improve adhesion of the coating. In recent developments, the copper foils also are micro-structured to improve the speed at which the electrolyte can be filled into each cell and to improve the wetting performance of the electrolyte to the electrode. The active materials are incorporated in a polymeric binder together with conductive additives. For optimum performance, the finished compound has to have a defined degree of porosity.
Because the foils are flexible, a key element in a pouch battery is the separator, which maintains a gap between the electrodes to prevent short circuits. The separator has to be structured to allow ions generated in the electrolyte to pass through it. Traditionally, the separator was a plastic film, around 25 microns thick, which is micro-porous. In this battery structure, the electrolyte is a lithium-based liquid compound.
Another battery structure, for polymer batteries, uses a gel as a separator. This gel also forms the electrolyte; however, because of the softness of the gel, this type of structure is particularly sensitive to metal-particle contamination.
More recent battery developments use a micro-porous, ceramic film either as a separator or more radically as a combined separator and electrolyte. The stronger ceramic reduces the risk of short circuits through piercing by metal particles.
Controlled porosity throughout the battery-cell structure is a key element in good battery performance. Pores blocked by contamination cause significant levels of defects.
Electrode-manufacturing process
Figure 2 shows a block diagram of a typical battery-manufacturing process.

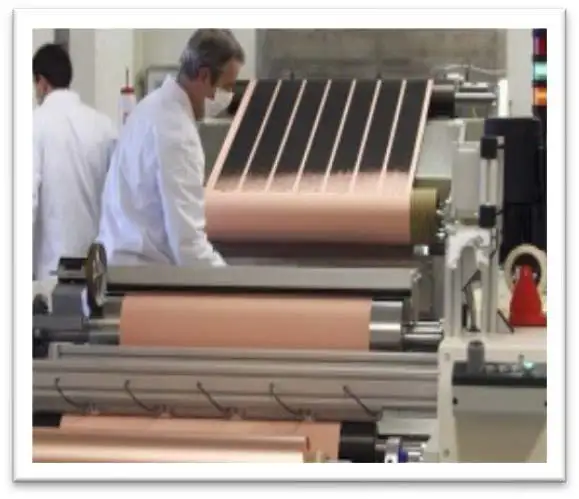
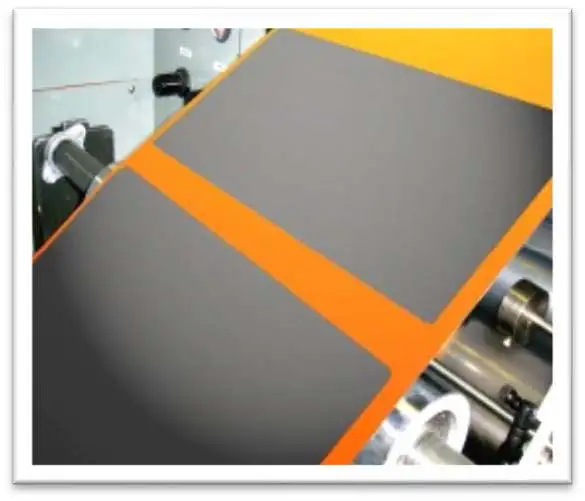
The most common method of applying the active-material matrix to the collector foils is by R2R coating. A variety of coating methods can be used with slot-die, and flexographic or gravure printing being the most common. The active-matrix deposition is patterned-coated as shown in Figures 3 and 4 to give the cell its conductive area while allowing an uncoated area on the sides for welding the cell together.
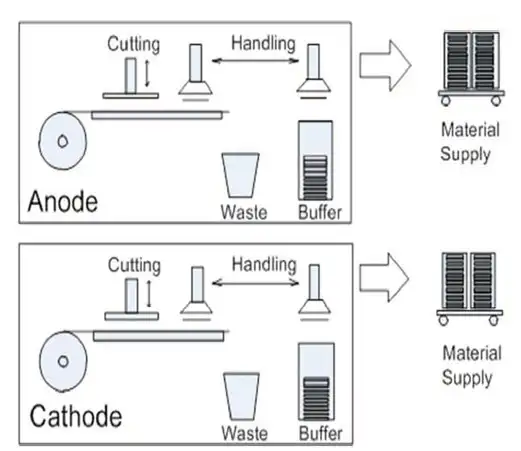
The coated foil then is dried in a vacuum oven to remove all traces of moisture that could react with the active materials to make them non-functional. The dried coating then is calendered under high pressure to control the porosity level and ensure even thickness.
As moisture is an issue in degradation of the active materials, new developments include the electrostatic application of dry active powder onto the charge-collecting foils. This powder is hot-calendered onto the foil to melt the binder and fuse the active matrix onto the foil. If the amount of binder in the dry powder matrix is too low, then poor adhesion can result in matrix particles becoming detached, which in turn results in conductive contamination, disturbing the charge transfer mechanism.
The electrodes then are cross-cut into sheets and stacked with the separators (see Figure 5). Increasingly, the electrodes are interleaved with the separators, still in roll form, by Z-folding (see Figure 6) before being welded around three sides. After filling with liquid electrolyte, the final side is welded.
Requirements for contamination removal
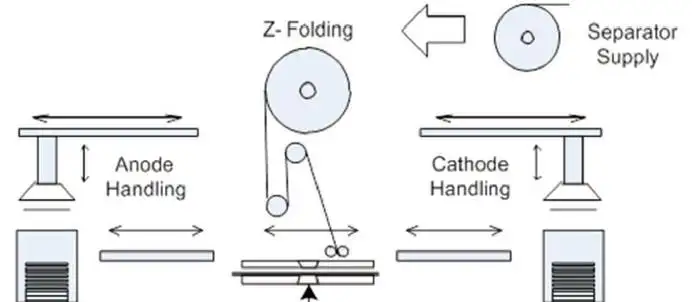
Although not all contaminants need to be removed at every stage in the battery-production process, it is necessary to investigate all potential sources of contamination throughout the R2R process to assess and eliminate both particulate and ionic contamination.
The risk of ionic contamination is mostly during the mixing of the chemicals that form the active matrices and the electrolyte, which should be supplied fully filtered and free of any water content. Care must be taken at the coating stage to prevent ionic contamination being incorporated into the active matrices at the coating head.
The key focus for reducing particulate contamination comes during electrode manufacture. Because moisture degrades the performance of the active matrices, much of the process has to be carried out in an extremely dry environment. This dry environment, in turn, results in very high static charges, which attract particles to the charged surfaces, making cleaning even more important. Contact cleaning is the preferred method of particulate removal for electrodes as it avoids any risk of moisture contamination that might come from an air-based system.
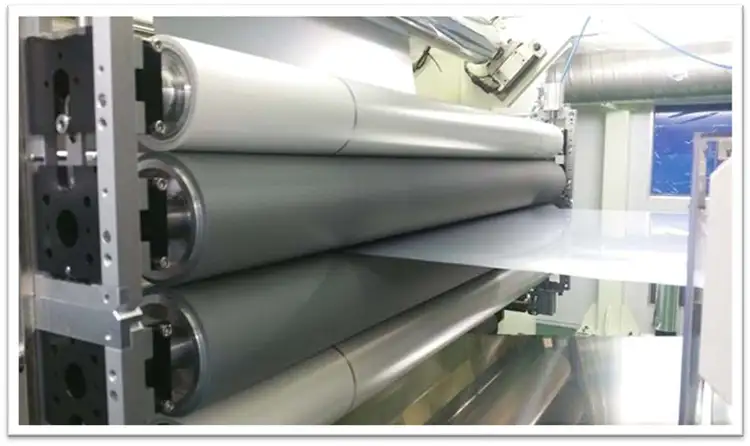
Cleaning of both sides of the electrode (see Figure 7) should always be carried out to ensure the film is completely clean throughout the process. There also is no risk of contamination from the backside of the film transferring onto the process rollers within the equipment.
A traditional place for cleaning in R2R processes is before coating, and this also is a key area for cleaning in battery production. Slitting of metal foils can generate particles of fine “swarf” with sharp ends. These constitute a high risk of penetrating the separator film and causing short circuits. This swarf from the initial slitting of the base foil must be removed before coating. In addition, after the electrodes are slit down to the cell width, they must be cleaned to avoid any contamination being trapped when the electrodes are laminated with the separators.
Any particles on the surface of the coated electrodes before calendering will be forced into the active matrix by the high pressure of the calender. This surface inclusion will affect the wetting of the electrolyte and inhibit charge transfer. Cleaning should be done on the dried coating before this process. Figure 8 shows this firm’s double-sided, narrow-web unit fitted to the inlet of a calender.
Both electrodes and the separator should be cleaned before stacking, winding up, interleaving or laminating to improve the performance of the battery.
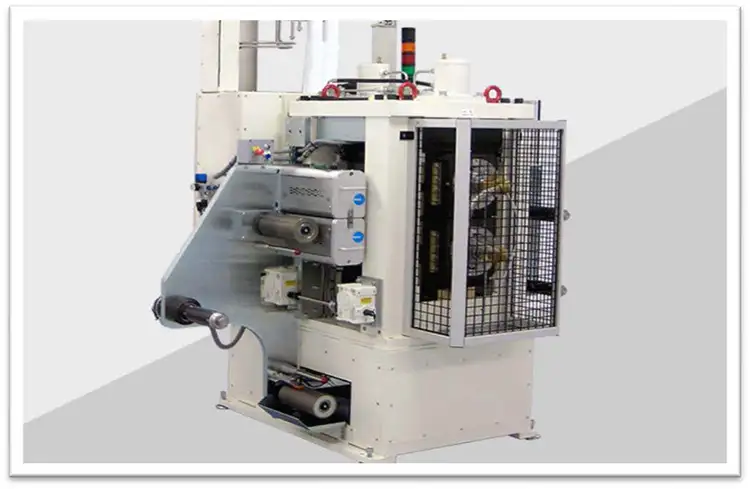
of a calender
A final area to consider in contamination control for battery manufacture is the process equipment itself. Transport rollers, in particular, can pick up contamination from the environment and from microslip on contact with the foils. This contamination can transfer onto the backside of the electrodes, causing defects in the subsequent processes. If calendering rollers have particles of contamination on them, they can impress the shape of the particle into the coating, thus reducing the active-matrix thickness and porosity level, while the backing rolls in the slot-die process can cause imprints on the collector foil itself. It is vital that these rollers are regularly cleaned and that the environment around them is enclosed and flushed with filtered air.
Conclusion
The process of manufacturing Lithium-ion batteries is very sensitive to contamination. Particulate and ionic contamination – and even water – can cause significant defects in the finished product. Worse still, these contaminants also can result in latent defects, which appear only during use by the end customer.
The electrode fabrication step is an area where contamination is a major risk. This process step contains many potential contaminants, such as particles from slitting and cutting the foils to metal debris from rollers and welding, all of which can cause short circuits. Environmental and other non-conductive particles can block pores in the charge transfer path reducing the performance of the finished battery.
Cleaning at all stages of the electrode-fabrication process can show significant benefits both to manufacturing yield and final product performance. For example, it has been shown that cleaning during electrode fabrication can reduce self-discharge defects by up to 40%, giving batteries a more consistent, longer life.
Sheila Hamilton, technical director for Teknek, Inc. (Inchinnan, UK) for 30+ years, has a Bachelor’s in Mechanical and Production Engineering from the University of Glasgow, together with an MBA from the University of Strathclyde in Scotland. The holder of numerous patents for technologies from electromagnetic interference (EMI) shielding and thermal management to various web-cleaning technologies, Hamilton has presented more than 70 technical papers at conferences around the world. At Teknek, she oversees development of key elastomer- and adhesive-roller technologies. Hamilton also is involved in several European-funded technology projects and works with many universities on development projects. She can be reached at +44-141-568-8100, email: sheilah@teknek.com, www.teknek.com.