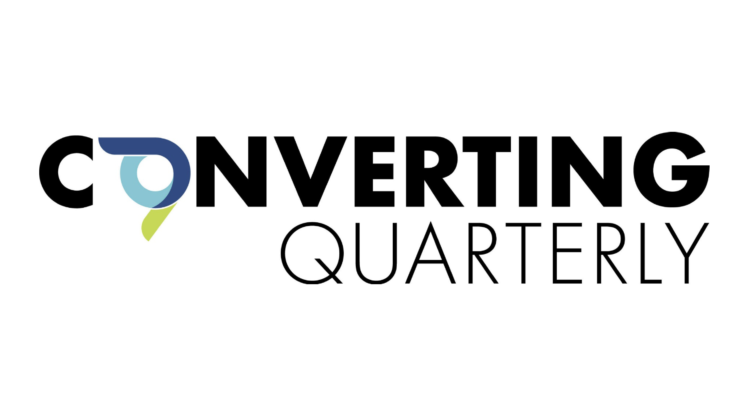
By Sage M. Schissel, Ph.D., applications specialist, PCT EB and Integration, LLC
Sustainable practices, including material reduction and the use of bio-based, recyclable or compostable materials, are forefront in the minds of both consumers and the packaging industry. This especially is true for flexible packaging, which traditionally has consisted of multilayer, non-recyclable structures. In this study, the use of electron-beam (EB) technology in the life cycle of a compostable, flexible package was investigated.
Editor’s Note: This technical paper originally was published in UV+EB Technology, 2020 Q2, pages 14-20. It since has been updated with newly expanded experimental-test results.
Introduction
In recent years, the packaging industry has experienced a consumer backlash regarding its use of plastics [1-3]. In response, companies have made sustainability pledges that include goals such as reducing their plastic consumption, reducing production waste and increasing the amount of recycled plastic in products [4-6]. These strides toward a more environmentally friendly future are commendable but are not always easily achieved. While consumers call for less single-use packaging waste, their expectations of how packaging performs – that food remains fresh, products remain undamaged and marketing remains eye-catching – have not waivered.
Electron beam (EB) technology is a multi-faceted tool that is well-suited for aiding the achievement of sustainability goals [7-12]. For instance, EB crosslinking can improve mechanical properties, allowing for reduced plastic consumption by downgauging film. It also can be used to increase compatibility between different polymers as well as virgin and recycled polymer blends. EB-induced chain scission can be used to recycle materials through degradation. Scrap polytetrafluroethylene (PTFE), for example, is irradiated to create micropowders that become ingredients in lubricants, inks and coatings.
Aiding the mono-material trend
In flexible-packaging production, EB is a useful tool in accomplishing one of the industry’s more recent trends – mono-layer and/or mono-material packaging. Traditionally, flexible packaging has relied on multilayer, non-recyclable structures to achieve the wide array of demands placed on a single pouch, from moisture and oxygen barriers to tear strength and sealability [13-14]. Mono-material structures are expected to meet these demands with a single type of plastic so that they more easily can be recycled or composted, but it is an ambitious challenge.
One way EB helps meet this challenge is by altering a polymer without any added chemistry. EB crosslinking and chain-scission provide property tuning that can be controlled through a selected substrate depth; a common example of this application is crosslinking an outer layer of polyethylene (PE) to increase heat resistance without affecting the sealing temperature of the inner PE layer. Additionally, EB can be used to polymerize inks and overprint varnishes (OPVs) without the need for initiators or solvents and with comparatively little energy [15-18]. OPVs are a sustainable alternative to lamination. Because inks and OPVs make up such a small fraction of the overall package, they’ve been shown to not hinder recyclability [19-20].
Accompanying the mono-material trend to make flexible packaging recyclable is a movement for compostable flexible packaging. Instead of relying on the ability to effectively repurpose packaging plastics, compostable packaging seeks to optimize the disposal of packaging waste by using biodegradable polymers. EB is less tested as a production solution in compostable packaging; however, there are several ways this technology may benefit the industry. Similar to recyclable packaging, it is expected that EB-cured inks and OPVs can be used without impacting the biodegradability of compostable packaging. Furthermore, EB could possibly result in accelerated disintegration if the packaging is exposed to high doses after consumer use.
Degradation by EB chain-scission
Molecular weight reduction by chain scission is a known effect of EB irradiation on cellulose, a common compostable material [21]. It is hypothesized that this degradation could be leveraged to decrease composting time. As compostable plastic packaging gains popularity, a reduction in the multi-week disintegration process may be imperative for the current infrastructure to keep up with increasing supply. According to a survey conducted by BioCycle in 2018, of the 185 identified food-waste compost centers in the US, only 53 reported being able to accept compostable plastics [22]. In addition, accelerating plastic disintegration should make it a more profitable enterprise; high volume turnover will help offset costs, such as sorting non-compostable plastics out of the feedstock.
In this study, the application of EB in the production and destruction of a mono-material, compostable, flexible food-packaging structure was investigated. Low doses of EB were used to cure matte and gloss OPVs on the compostable film, which then was tested to determine if the OPV inhibited or impeded compostability. Moreover, high doses of EB were used to induce chain-scissioning in the packaging structure (film/ink/EB-cured OPV) to ascertain whether such degradation at the end-of-life could efficiently reduce compost times. Puncture strength was measured as a means of analyzing the EB degradation.
Experimental
Materials: The substrate material used in this study was NatureFlex™ NK 120-gauge (NK120, Futamura) [23]. NK120 is a transparent cellulose film coated with PVdC for moisture and gas-barrier properties. It was selected because it is suitable both as a laminate and for mono-layer, flexible-packaging applications. NK120 is certified as industrial- and home-compostable. The primer used was DigiPrime® 050 (Michelman).
The ink used was the CMYK Indigo ElectroInk digital ink set (Hewlett-Packard) [20]. These digital inks are indirect food-safe and certified as industrial and home compostable [24-25]. In addition, the offset digital-printing process has some environmental advantages over analog methods, including less material waste, low energy consumption and no printing plates or cylinders.
The overprint varnishes (OPVs) used in this study were EHG-2601 (EG, DBT Coatings) and EMQ-3710 (EM, DBT Coatings) with high gloss and matte finishes, respectively [26-27]. These EB-curable OPVs were chosen because they are used in the flexible food-packaging industry and are formulated to protect and highlight the digital inks. Both OPVs meet ultra-low migration standards, are indirect-food-contact safe and are free of initiators and solvents.
Methods: Sample Preparation: NK120 film was coated with primer and printed using an HP Indigo 20000 digital press. Unprinted NK120 film also was used for some samples. Both the printed and unprinted films then were corona-treated at 13.8 W/in, OPV was applied with an indirect gravure coater, and lastly the OPV was cured at 30 kGy and 115 kV using a Broadbeam EP electron-beam pilot line (PCT EB and Integration). Oxygen levels were kept to <200 ppm using a flow of 99.999% pure N2 in the beam. The EM OPV was applied using a 5-BCM, 400-line ceramic anilox with a resulting coatweight of approximately 2.0 gsm. The EG OPV was applied using a 10-BCM, 200-line steel anilox with a resulting coatweight of approximately 3.5 gsm.
Additionally, some samples were exposed to EB for a second time for a post-treatment, which was intended to reduce composting time through degradation of the cellulose film. The post-treatment was done in air, at 200 kV, 50 fpm, and at doses ranging from 50 to 400 kGy. A level of 200 kV was chosen as an accelerating voltage to ensure an equal-dose distribution through the complete thickness of the film.
Compostability Testing: To determine the influence of the samples on the composting process, samples were composted by Organic Waste Systems (OWS) following the test method detailed in ISO 16929:2013 [28]. The pilot-scale aerobic composting test consisted of organic biowaste (a mixture of vegetable, garden, and fruit waste) in a 200-L composting bin monitored through temperature and exhaust-gas composition for 12 weeks. The mixture was turned by hand every one to two weeks. For the test to be deemed valid, the temperature must remain between 60 to 75° C during the first week and below 65° C thereafter, with the minimum temperature remaining above 40° C for at least four consecutive weeks. Photographs were taken on a weekly basis to visually evaluate the percentage of disintegration the sample material had undergone. Because of this qualitative evaluation metric, the results of this compostability testing serve as an indicator only of whether the sample will pass a quantitative (mass balance) test. The unprinted samples tested for compostability are listed in Table 1 and the printed and post-treated samples are listed in Table 2.
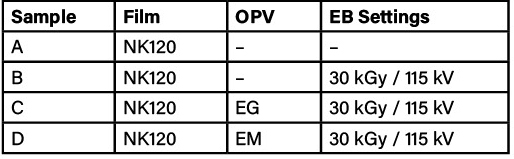

Puncture Testing: Puncture resistance was used as a measure of film strength after EB exposure. Squares measuring 6.5-in. of sample material were held taut in a fiberglass board frame (Micarta) using a rubber O-ring with a 5-in. outer diameter and 0.210-in. width. The frame had a 4-in.-diameter circular window exposing the sample. A compressive load was applied to the center of the exposed sample at a rate of approximately 3 to 4 lb/s using a rounded probe with an arc 1.094 in. wide and 0.270 in. high. A broad probe was chosen to gain better resolution of the film strength lost at varying EB doses. The applied load was measured using a Uline platform dial scale (Model No. H-176). The recorded value was taken as the maximum load applied before the sample ruptured, and the probe was able to push through the sample. For each sample condition, five repetitions were completed and the values reported are an average of those repetitions. The error reported is the standard deviation of the five repetitions.
Editor’s Note: Part 2 of this technical paper will cover the experimental results and discuss packaging compostability, visual effects, puncture-strength degradation and compost results.
Acknowledgements
The author would like to acknowledge DBT Coatings for initiating and funding the first round of compostability testing and sharing the results. These data provided the impetus for further exploration of EB’s role in compostable packaging. The author also would like to acknowledge Futamura for donating the NK120 film and GOpak for donating printing-press time and materials.
References
- Crawford, E., 2019. Consumer push-back against plastic will spur manufacturers to seek new packaging in 2019. https://www.foodnavigator-usa.com/Article/2019/01/22/Consumer-push-back-against-plastic-will-spur-manufacturers-to-seek-new-packaging-in-2019
- Pierce, L. M., 2019. Consumers want non-plastic packaging options. https://www.packagingdigest.com/sustainable-packaging/consumers-want-non-plastic-packaging-options-2019-11-14
- Heidbreder, L. M., Bablok, I., Drews, S., Menzel, C., 2019. Tackling the plastic problem: a review on perceptions, behaviors, and interventions. Sci. Total Environ. 668, 1077-1093.
- Nestlé. What is Nestlé doing to tackle plastic packaging waste. https://www.nestle.com/ask-nestle/environment/answers/tackling-packaging-waste-plastic-bottles
- Unilever. Rethinking plastic packaging – towards a circular economy. https://www.unilever.com/sustainable-living/reducing-environmental-impact/waste-and-packaging/rethinking-plastic-packaging/
- Pepsico, 2018. Pepsico’s work to improve recycling in the U.S. https://www.pepsico.com/docs/album/esg-topics-policies/pepsicoworktoimproverecycling.pdf?sfvrsn=3071b156_12
- Burillo, G., Clough, R. L., Czvikovsky, T., Guven, O., Le Moel, A., Liu, W., Signh, A., Yang, J., Zaharescu, T., 2002. Polymer recycling: potential application of radiation technology. Radiat. Phys. Chem. 64, 41-51.
- Czvikovsky, T., 1995. Reactive recycling of multiphase polymer systems through electron beam. Nucl. Instrum. Meth. B. 105, 233-237.
- Clough, R. L., 2001. High-energy radiation and polymers: a review of commercial processes and emerging applications. Nucl. Instrum. Meth. B. 185, 8-33.
- Mészáros, L., Bárány, T., Czvikovsky, T., 2012. EB-promoted recycling of waste tire rubber with polyolefins. Radiat. Phys. Chem. 81, 1357-1360.
- Navratil, J., Manas, M., Mizera, A., Bednarik, M., Stanek, M., Danek, M., 2015. Recycling of irradiated high-density polyethylene. Radiat. Phys. Chem. 106, 68-72.
- Jamdar, V., Kathalewar, M., Dubey, K. A., Sabnis, A., 2017. Recycling of PET wastes using electron beam radiations and preparation of polyurethane coatings using recycled material. Prog. Org. Coat. 107, 54-63.
- Wagner, J. R. (Ed.), 2016. Multilayer flexible packaging. Elsevier.
- Morris, B. A., 2016. The science and technology of flexible packaging: multilayer films from resin and process to end use. William Andrew.
- Mehnert, R., 1995. Electron beams in research and technology. Nucl. Instrum. Meth. B. 105, 348-358.
- Golden, R., 2012. What’s the score? A method for quantitative estimation of energy use and emission reductions for UV/EB curing. Radtech Report. (3), 44-48.
- Golden, R., 2008. Sustainability advantages of ultraviolet and electron beam curing. Radtech Report. (4), 43-47.
- Sanders, R., 2006. Electron beam: one way to mitigate rising energy costs. Radtech Report. (2), 20-22.
- Korn, D. J., 2005. Recyclability of UV and EB printed and coated paper. Radtech Report. (3), 47-49.
- HP, 2018. HP Indigo electroink: frequently asked questions. https://www8.hp.com/h20195 /v2/ GetPDF.aspx/4AA7-4166ENW.pdf
- Driscoll, M., Stipanovic, A., Winter, W., Cheng, K., Manning, M., Spiese, J., Galloway, R. A., Cleland, M. R., 2009. Electron beam irradiation of cellulose. Radiat. Phys. Chem. 78, 539-542.
- Goldstein, N., 2018. Quantifying existing food waste composting infrastructure in the U.S. http://www.biocycle.net /pdf/2019/FoodWasteCompostInfra.pdf
- Futamura. NatureFlex™ NK technical data sheet.
- HP, 2016. HP indigo for food packaging printing: regulatory overview. https://www8.hp.com/h20195 /v2/GetPDF.aspx/4AA4-8153ENW.pdf
- Gilbert, N., 2019. HP indigo digital print certified for compostable packaging. https://press.ext. hp.com/us/en/press-releases/2019/hp-indigo-digital-print-certified-for-compostable-packaging.html
- DBT Coatings. Technical data sheet EHG-2601 EB high gloss top coat: low extractables and low migration.
- DBT Coatings. Technical data sheet EMQ-3710 EB low gloss top coat: low extractables and low migration.
- ISO 16929:2013. Plastics – determination of the degree of disintegration of plastic materials under defined composting conditions in a pilot-scale test. https://www.iso.org/standard/62948.html
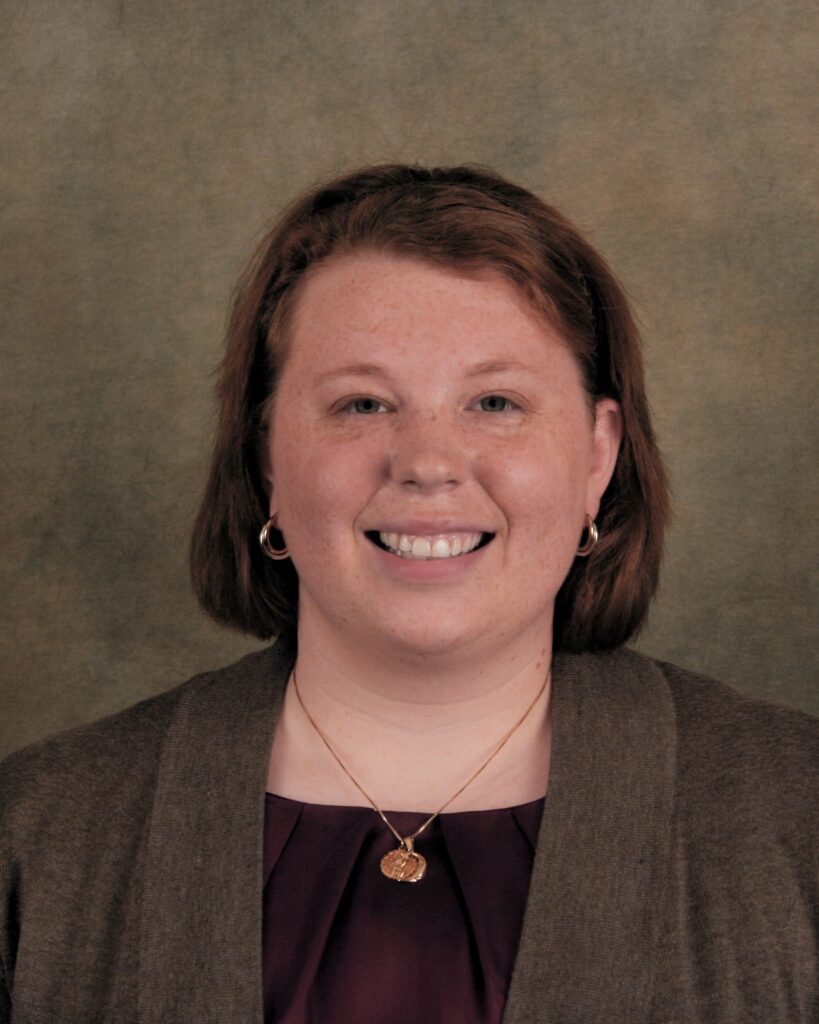
Sage Schissel, an applications specialist at PCT EB and Integration (Davenport, IA), holds of Bachelor’s degree in Engineering Science from Wartburg College, and a Ph.D. in Chemical and Biochemical Engineering from the University of Iowa. She introduces customers to electron-beam technology and works with them to enhance existing processes by using EB or to develop entirely new processes and applications. Sage has been involved in EB research for the past 10 years. She can be reached at 563-285-7411, ext. 4429, Sage.Schissel@pctebi.com, www.pctebi.com.