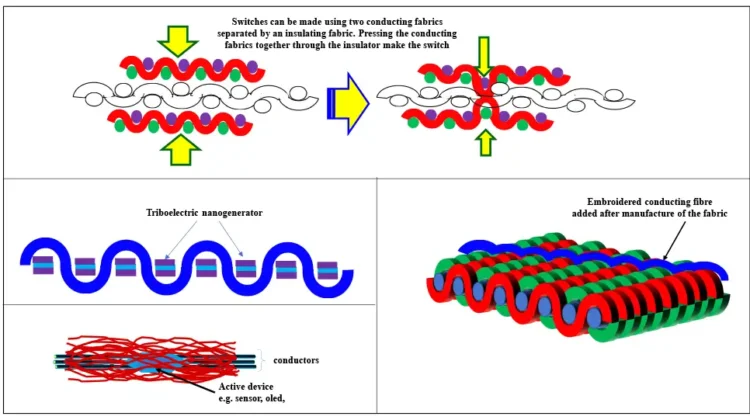
By Charles A. Bishop, Ph.D., C.Eng., principal., C.A.Bishop Consulting, Ltd.
Abstract
It is something of an adventure to manufacture flexible electronics, but imagine the additional challenge of making the devices capable of passing through a washing machine multiple times while still remaining functional. This is what is expected of flexible electronics that are integrated into textiles and clothing. Early examples had the flexible electronics just stuck onto the clothing, but these had limited lifetimes and were not able to be washed. The next step was to print the electronics directly onto the fabric, usually using a first planarizing coating, which was little different from gluing devices onto the fabric. Since then, there have been developments in producing fibers that are photovoltaic, piezoelectric, supercapacitors or simply conducting. This has enabled fabrics to be manufactured that can generate power and store the energy, as well as be integrated with sensors to provide functional clothing. In this paper, I will highlight some of the challenges in converting printed-electronics fibers or yarns into clothing.
Introduction
Flexible electronics cover a huge range of disparate products, from flexible displays, photovoltaics, batteries, supercapacitors, electrochromics, circuits, radio frequency identification (RFID) tags and sensors to piezoelectric and triboelectric energy harvesters and electromagnetic / radio frequency scavenging. The substrates for such a wide range of products also are diverse and include metal foils, glass, ceramics, polymer films, paper and fibers (both woven and non-woven fabrics). Of these, perhaps the most challenging is to produce flexible electronics that not only function well but also are aesthetically pleasing and then integrate them into clothing [1-4]. This is hard enough but making the whole system robust enough that it then can be machine-washed multiple times and still work must have made the design more challenging. Perhaps the mobile phone industry could learn from the fashion industry and produce a mobile phone that can be left in a pocket and pass through a washing machine without damage.
There are many different types of flexible electronics that can be integrated into clothing. In medical and sports clothing, there are sensors that monitor heart rate, perspiration rate, temperature and breathing [5-8]. These sensors then need to either store and periodically download the data or, using WiFi, send the data to a remote location. To manage and control functions, it is useful if there is a source of power, and so fabrics have been produced for energy harvesting [9-12]. This may be done using an aerial to collect energy from some nearby source (RF scavenging), or it may make use of movement by the wearer of the clothing. Piezoelectric materials generate power that then may be stored using other parts of the fabric that have supercapacitor structures coated on the fibers [13]. Using a layered-material construction, touch switches also have been woven into the clothing, enabling devices to be switched on and off [14].
Challenges and choices
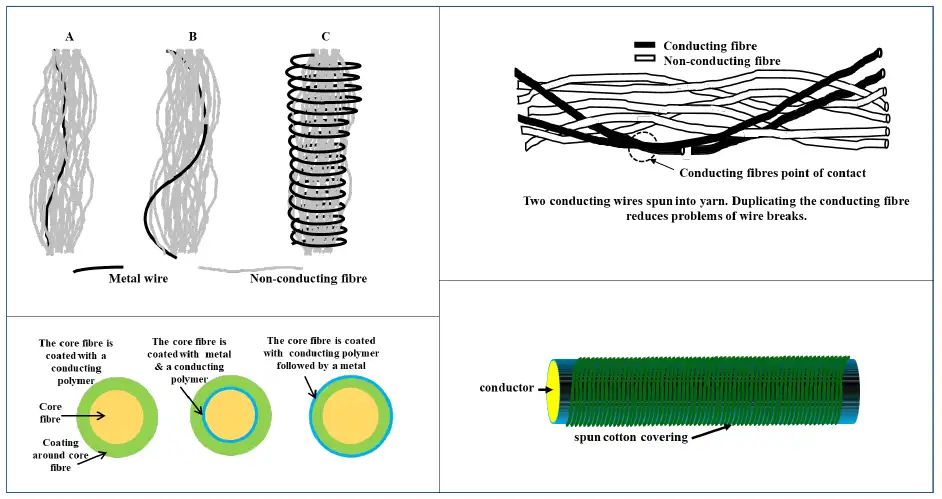
It is worth having a look at some of the challenges and choices that arise in developing flexible electronics in clothing. A starting point is producing conducting tracks in a garment (see Figure 1). This can be achieved by simply weaving in conducting metal wires, spinning wires into spun fibers, coating the woven or knitted material, coating the fibers or making the fibers out of intrinsically conducting polymers – or a combination of these methods.
Using metal wires has the advantage that the conductivity is good, but there is the disadvantage that the fine wires can fail with too much bending. The fine wire may be covered with cotton twisted around the metal to hide the metal [15] and provide a surface that could be dyed, as well as providing a more comfortable feel to the fabric. To offset the problem of metal breaking, duplication of wires can be done, but the result is the clothing will become stiffer because of the metal being less flexible than the rest of the fibers.
Coating the fibers can be done a number of different ways. The finished clothing can be printed with a conducting ink or coating, but this changes the texture of the material – making it stiffer and also more noticeable and usually aesthetically less appealing. Coating the fibers before weaving or knitting the fabric can overcome this. Coating the fibers with metal can cause a problem with degradation as the metal coating may break when the fibers are stressed. This can be overcome by coating the fibers with a conducting polymer. The conductivity of the conducting polymers may be lower than desired and so coating the fibers with conducting polymer and then metallizing the surface improves the conductivity over just using a conducting polymer and, even if the metal coating is cracked, the underlying polymer will bridge the gap and so the loss of conductivity is limited.
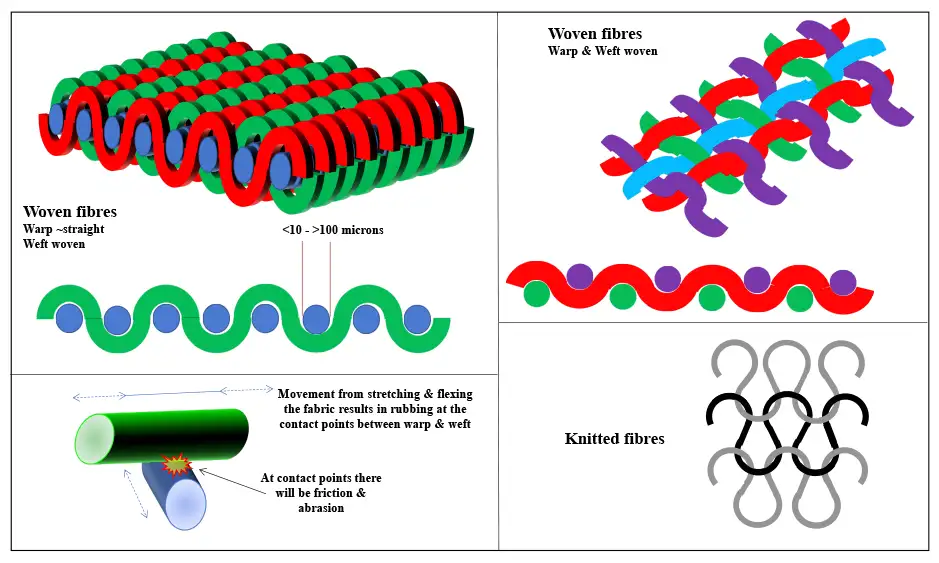
As with any coating process, the adhesion is important, particularly when we look at the stress that the fiber or yarn is subjected to when making it into a woven cloth or knitted fabric. If the fibers being woven are 50 microns in diameter, the bend on the fibers will be around a 25-micron radius, and the bend will be on the order of 180° for woven fabric or closer to 270° when knitted. This is much more challenging than the radius of a few millimeters that many flexible electronics are subjected to. This higher stress makes high adhesion crucial to the fabric being robust. The fibers are in direct contact with other fibers after weaving or knitting and, to enable the fabric to be flexible and have good drape, the fibers have to move relative to each other. This adds abrasion to the list of degradation mechanisms to the fiber surfaces. This is shown in Figure 2, along with some simple woven and knitted structures.
Withstanding the spin cycle
Designers were among the first to work with flexible electronics for clothing, with apparel containing electroluminescent fibers, switchable lights, fabric keyboards, simple-computing and sound-activated color-changing fabrics. Models walking down catwalks brought plenty of attention to the potential for flexible-electronics fabrics, but these demonstration items were not expected to be worn on many occasions or washed. To convert the fabrics to ones able to be used by the wider public required the devices to be robust enough to withstand much more flexing, as well as regular washing including by washing machines.
Work has been done on a variety of different types of conducting fabrics, including those incorporating wires, conducting polymer-coated fibers and printed conducting tracks onto fabrics [16-19]. It is not only the problem of chemical attack or mechanical failure of the conducting fibers that creates failure but also the contact points where the fiber has to be attached to the active device, sensor or power source [20]. One of the additional concerns that has been raised is that, where silver is used to make the conducting fibers, some of the silver may be lost during washing. If the silver ink or coating used contains silver nanoparticles, it can become a source of environmental contamination [21-22], which may restrict the use of this type of conductor. Passing clothing through a washing machine also provides an aggressive stress test of any coating and reinforces the necessity of any coating having very high adhesion to the fiber core.
From fiber to fabric to garment
![FIGURE 3. Schematics of four different methods of adding functionality to fabrics. Top shows the use of layers of fabric where the top and bottom layers are conducting, which can be made to contact each other by pressing the two conducting layers together by distorting the insulating middle layer. Middle left shows a flat tape triboelectric nanogenerator Warp that is not woven with the Weft fibers woven around the Warp [24]. Bottom left shows how an active device, such as a sensor, can be incorporated into a bundle of fibers. The fibers are spun around the conductors and device so that it is hidden from view and protected. On the bottom right, the schematic shows an embroidered track that has been added to the basic fabric sometime after the fabric has been manufactured [25].](https://convertingquarterly.com/wp-content/uploads/2025/02/cq420pfe-bishop_FINAL_3.webp)
Fabricating clothing by traditional methods is done by producing panels of fabric that are sewn together to make the completed garment. This means that as the panels are combined the conducting fibers have to be connected. Connecting conducting fibers can be done with many of the same methods used for other electronic devices, such as soldering, brazing, welding, crimping and adhesive-bonding with conducting-polymer adhesive (see Figure 3). In addition, sewing with a conducting fiber is possible, although the resistivity can be variable and prone to loss of conductivity over time [23].
Often, the connections are hidden in seams, collars or pockets and may be protected using a polymer tape or a soft polymer encapsulant, such as silicone or polyurethane, any of which may smooth out the joint. Alternatively, if the joint is small enough, it may be possible to fit it into a button, providing it can be suitably located on the garment. As the joint makes the connected area stiffer and so more prone to failures under repeated bending, it usually is aimed for the joints to be located in areas where the normal flexing of the garment is low.
Conducting tracks may be added after the garment is completed by embroidering the tracks onto the surface of the garment. The embroidery may be in straight lines but, if it is done as a zig-zag or serpentine shape, it can reduce stress when the garment is stretched, thus reducing the possibility of the conducting wire or fiber breaking.
A 3D option for conductive fabrics
More recently, progress has been made in the 3D knitting of garments. This technology is based on knitting tubes rather than flat sheets. Imagine a dress starting off as three tubes, for the two arms and the body, and shaped and brought together to be merged into one tube. The spacing of the stitches can be varied to change the size of the tubes, so the garment may be customized to fit the exact shape of the person it is made for. With this technology the texture, shape, colors and functionality can be varied, so instead of assembling different panels of fabric, a garment may be knitted as a single piece. This helps minimize the number of electrical joints that have to be made.
A good example of this technology is a balaclava developed for athletes that has electrical heating around the nose and mouth to reduce the risk of chest infections in winter [26-27]. This particular item uses a battery to power the heating wires, with the balaclava containing a pouch for the battery with the connector wires terminating in a connector to fit the battery. Also included in the design was the use of reflective fiber to make the user more visible at night. The balaclava is knitted to be a close fit to the skull and neck, with the knitting structure allowing for the neck section having a large amount of stretch to allow for pulling it over the head and still fitting close to the neck after stretching.
A video description of the basics of the 3D-knitting process is available on YouTube [28].
Conclusion
Flexible electronics in textiles have moved forward quite rapidly over the last few years, with medical, military and elite sports leading many of the developments. This has been aided by the progress in 3D-knitting machines. This progress is tempered by the cost of the individual garments, which still is high. It is expected that the cost per garment gradually will decline as further improvements are made in the 3D-knitting machines, enabling the garments to be available to a much wider section of the populace. Garments with functional electronics still have some way to go before they become robust enough to withstand many 10s of cycles through washing machines, but this too is improving. Much of this is because more ways of encapsulating wires, fibers, yarns and devices have been developed, as well as methods of protecting the electrical contacts. This harsher use of flexible electronics is worth watching as some of the techniques might help the less onerous applications, such as flexible displays and photovoltaics.
References
- Cherenack, K. & Van Pieterson, L. Smart textiles: Challenges and opportunities. J. Appl. Phys. 112, (2012).
- Chen, J., et al. Micro-cable structured textile for simultaneously harvesting solar and mechanical energy. Nat. Energy 1, 16138 (2016).
- Jost, K., Dion, G. & Gogotsi, Y. Textile energy storage in perspective. J. Mater. Chem. A 2, 10776–10787 (2014).
- Zhang, Z., et al. Weaving efficient polymer solar cell wires into flexible power textiles. Adv. Energy Mater. 4, 1–6 (2014).
- Coyle, S., et al. Smart Nanotextiles: A Review of Materials and Applications. MRS Bull. 32, 434–442 (2007).
- Simon, C., Potter, E., Mccabe, M. & Baggerman, C. Smart Fabrics Technology Development – Final Report. (2010).
- Fabrice Axisa, Pierre Michael Schmitt, Claudine Gehin, Georges Delhomme, Eric McAdams, and André Dittmar Flexible Technologies and Smart Clothing for Citizen Medicine, Home Healthcare, and Disease Prevention IEEE Trans on Info. Tech. in Biomedicine VOL. 9, NO. 3, Sept 2005 pp 325- 326
- Bartels T.V. Handbook of Medical Textiles, Cambridge, Woodhead Publishing 2011.
- Farmer, J. R. A comparison of power harvesting techniques and related energy storage issues by A comparison of power harvesting techniques and related energy storage issues. Mechanical Engineering 135, (Virtginia Polytechnic Institute and State University, 2007).
- Zhou, T. et al. Woven structured triboelectric nanogenerator for wearable devices. ACS Appl. Mater. Interfaces 6, 14695–14701 (2014).
- Lemey, S., Declercq, F. & Rogier, H. Textile antennas as hybrid energy-harvesting platforms. Proc. IEEE 102, 1833–1857 (2014).
- Yildiz, F. Potential Ambient Energy-Harvesting Sources and Techniques. J. Technol. Stud. 40–48 (2007). doi:10.21061/jots.v35i1.a.6
- David Harrison, Fulian Qiu, John Fyson, Yanmeng Xu, Peter Evans and Darren Southee A coaxial single fibre
supercapacitor for energy storage. Phys. Chem. Chem. Phys., 2013, 15, pp 12215–12219 - Seiichi Takamatsu, Takahiro Yamashita, Takahiko Imai and Toshihiro Itoh. Fabric touch sensors using projected self-capacitive touch technique. Sensors & Materials, Vol.25, No.9, (2013) pp 627-634
- Ashok Kumar L Study on Different Techniques of Fabricating Conductive Fabrics for Developing Wearable Electronics Garments. J Textile Sci Eng 2015, 5: 212. doi:10.4172/2165-8064.1000212
- Xuqing Liu, Ruisheng Guo, Yuxi Shi, Libo Deng, Yi Li. Durable, Washable, and Flexible Conductive PET Fabrics Designed by Fiber Interfacial Molecular Engineering Macromol. Mater. Eng. 2016, 301, pp 1383-1389
- Umut Kivanc Sahin and Senem Kursun Bahadir Effects of Home Laundering on Electrical Resistance of Signal Transmission Lines on Colored E-Textiles, Journal of Energy and Power Engineering 11 (2017), pp 336-344
- Ozan Kayacan Comparative study about the effect of cleaning processes on the transmission performance of textile based conductive lines. Industria Textila˘ ISSN 1222–5347, 2015, vol. 66, nr. 4 pp 176-183
- Bangting Wu1, Bowu Zhang, Jingxia Wu, Ziqiang Wang, Hongjuan Ma, Ming Yu, Linfan Li & Jingye Li. Electrical Switchability and Dry-Wash Durability of Conductive Textiles Sci. Rep. 5, 11255; doi: 10.1038/srep11255 (2015).
- I. Kazani, F. Declercq, M. L. Scarpello, C. Hertleer, H. Rogier, D. Vande Ginste, G. De Mey, G. Guxho, L. Van Langenhove. Performance study of screen-printed textile antennas after repeated washing. AUTEX Research Journal, Vol. 14, No 2, June 2014, pp 47-54
- Washing nanotextiles: can nanosilver escape from clothes?European Commission DG ENV News Alert, Issue 178. December 2009.
- Geranio, L., Heuberger, M., Nowack, B. The Behavior of Silver Nanotextiles during Washing. Environmental Science and Technology. 43(21), 2009, pp 8113-8118
- I. Locher. Joining technologies for smart textiles. Chapter 10, section 10.5 Overview of existing jointing technologies in the electronics and in the textile world. In book – ‘Multidisciplinary Know-How for Smart Textiles Developers’ Ed.Tunde Kirstein (Woodhead Publishing Series in Textiles) ISBN-13: 978-0857093424 2013
- Jun Chen, Yi Huang, Nannan Zhang, Haiyang Zou, Ruiyuan Liu, Changyuan Tao, Xing Fan and Zhong LinWang. Micro-cable structured textile for simultaneously harvesting solar and mechanical energy. Nature Energy Article No. 16138, DOI: 10.1038/NENERGY. 2016.138.
- Asimina Kiourti and John L. Volakis. Colorful Textile Antennas Integrated into Embroidered Logos. J. Sens. Actuator Networks. 2015, 4, pp 371-377 doi:10.3390/jsan4040371
- Nottingham Trent University Electric balaclava to avert chest infections in cold weather (2016, November 24) retrieved 23 July 2020 from https://phys.org/news/2016-11-electric-balaclava-avert-chest-infections.html
- http://nfc.stoll.com/video/stoll_performance_plus.mp4
- WholeGarment – The Revolution Continues. https://www.youtube.com/watch?time_continue=109&v=kZE8rvPYbII&feature=emb_logo
Dr. Charles A. Bishop holds a Bachelor’s degree in Materials Engineering with a Diploma in Industrial Studies. His research led to developing a process for manufacturing titanium-based bone implants for tendon location. He went on to obtain a Master’s degree and Ph.D. following further research into vacuum-deposition processes. Bishop has more than 35 years of experience in vacuum deposition, mainly onto flexible webs. He has published two books, writes the “Vacuum Verbiage” Q&A technical column for this publication and moderates the online “Vacuum Web Coating” Technical Channel. Bishop can be reached at +44-1509-502076, email: [email protected].