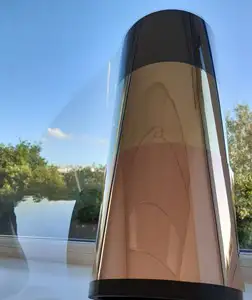
By Dermot Monaghan, Victor Bellido-Gonzalez, Tommaso Sgrilli, Patrick McCarthy and Lara Maroto Diaz of Gencoa, Ltd., and Oihane Hernandez-Rodriguez of Fundación Tekniker, Eibar, Spain
A novel human coronavirus that now is named severe acute respiratory syndrome coronavirus 2 (SARS-CoV-2 (formerly called HCoV-19) emerged in Wuhan, China, in late 2019 and now is causing the COVID-19 global pandemic. Until widespread vaccination, there is a strategic need to invest in technological solutions to reduce and slow the impact of the pandemic. The SARS-CoV-2 virus can be present on plastic and glass surfaces for several days. Once a hand has touched a contaminated surface, hand-washing reduces the probability of infection by removing the virus. Gloves reduce the chance of contagion if changed frequently, although the virus will be transferred by a gloved hand to the next surface. Once travel restrictions are relaxed, the chance of spread from surfaces will increase as the population begins to move around again. Vacuum coating has a solution that can be used to greatly reduce the spread from commonly touched surfaces. Vacuum-deposited, copper-based, nanostructured films are highly biocidal and create self-sanitizing surfaces. These films have a viral- and microbial-killing effectiveness above conventional cleaning methods. The killing action is continuous; hence, it will have a positive impact to reduce the probability of cross-contamination from surfaces. Tests show complete killing of 1 million coronavirus particles placed on such surfaces within 15 mins.
This paper presents a solution against all forms of infection derived from touch surfaces by using a nanotechnology coating-based adhesive pad to protect the public from cross-contamination. The coating development has been focused on flexible and transparent substrates which can be easily upscaled using roll-to-roll (R2R) vacuum-production techniques. Different biochemical and biological tests have been performed to quantify the biocidal activity.
Editor’s Note: This paper is based on a presentation at the 2020 AIMCAL R2R USA / SPE FlexPackCon® Virtual Conference, held online in October 2020. Part 1 addressed touch surfaces as a source of contagion, copper as a biocidal material and anti-bioactivity-coating deposition.
Production of copper-based coatings
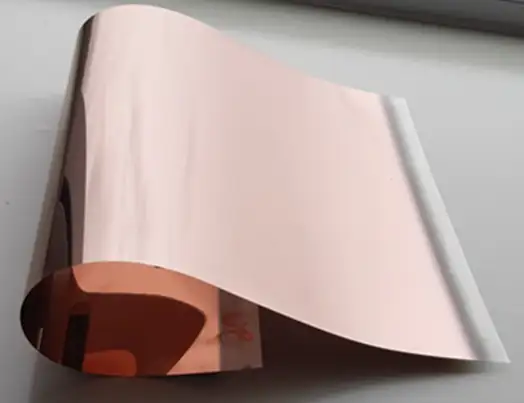
Sputtering copper onto surfaces is relatively straightforward as the material is quick to deposit (high sputter yield) and the high thermal conductivity of sputter targets allow high powers to be applied. Copper can be thermally evaporated but typically is not as easy as aluminum-based evaporation. Analysis of sputtered and thermally evaporated copper onto plastic web demonstrates that both methods can produce highly biocidal surfaces.
As explained, copper films require the correct surface structure to be active. It is apparent that very smooth copper surfaces are not biocidal initially. This is a surprising result and has been reproduced a number of times. Copper ions are toxic, but the effective release of copper ions from a surface also is dependent on the surface roughness. Copper deposited with the right energy conditions on a silicon wafer forms a continuous film with little growth structure – essentially featureless. These coating types do not display the mechanisms required to produce bioactivity. However, the very smooth surfaces can be “activated” by chemical etching or ion surface treatment to create some surface variations that drive the mechanisms needed for bioactivity. This has been demonstrated by tests on commercial copper-adhesive tapes. Initially, these surfaces are not active; however, with “aging” they can develop surface oxides, and the surfaces begin to be biocidal. In this respect, the newly exposed “clean” copper tape is less desirable than the more “dirty”-looking oxidized form. It would appear that smooth surfaces in a uniform electrical state are not effective. Some disturbance to this uniform surface is required to drive a reaction to disturb microorganisms and cause cell death.
Creating copper layers by sputtering or evaporation on a plastic film on a roll-to-roll (R2R) format (see Figure 6) naturally leads to the creation of the correct growth structure for bioactivity. This is due to the 20- to 30-nm size growth features that result, which lead to the “supercharging” surface effect when compared to conventional thermomechanical production methods for copper. Hence, while standard copper surfaces have a LOG 3 (99.9%) killing effect, thermally evaporated layers operate to LOG 4-5, and sputtered layers to LOG 5-6. This means that vacuum deposition is the best method to create copper-based biocidal surfaces. The other advantage to the vacuum-coating process is reduced material use and hence lower cost. Biocidal surfaces require activity only at the surface region, so the bulk of the substrate material is not active. Vacuum coating applies a thin layer (5 to 100 nm) on a surface, and when this is compared to the thinnest form of bulk copper (35-micron-thick copper-adhesive tape), more than 300X less copper material is used.
To easily fix such copper-based protection onto a surface, an adhesive backing is required, which also allows removal in the future. Copper vacuum-coated plastic will be the most effective surface protection available. No other option will be as aggressive or as low-cost. The protection will last months / years, and although discoloring will detract from the surface appearance, it actually will be no less biocidally effective. Alloys of copper can be used to change the color and provide better, longer-term appearance and wear resistance.
The production of transparent coatings
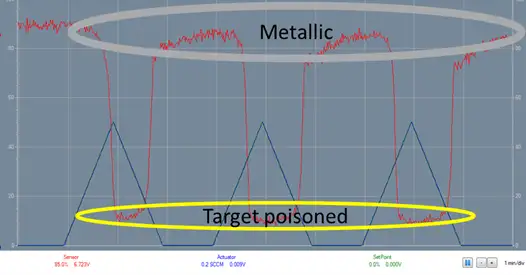
The transparent coatings can be produced using reactive magnetron sputtering, which introduces a gas into the metal vapor flux to form a compound. This method is used widely to create a range of metal-oxide layers to produce optical effects on surfaces, such as anti-reflection or solar-control. The reactive-sputtering process is, however, highly unstable when operated in what is known as the transition mode, maintaining the sputter target in metallic surface condition.
Transition mode of operation is desirable as the deposition rates are 2X to 3X faster and optical properties easier to tune. This firm’s fast-feedback controller combined with our new gas sensor (as the input signal) provides an automated-control solution to allow operation in the unstable region [14,15]. The controller adjusts the composition and transparency of the films by varying the metal-to-gas ratios. The control value is selected from the hysteresis behavior of the reactive system somewhere between the metallic and poisoned states (see Figure 7).
The combination of copper-sputtering with oxygen-gas feedback control produces a transparent form of copper which can be applied readily to plastic or glass surfaces.
Biochemical method for assessment of biocidal activity of vacuum-deposited coatings
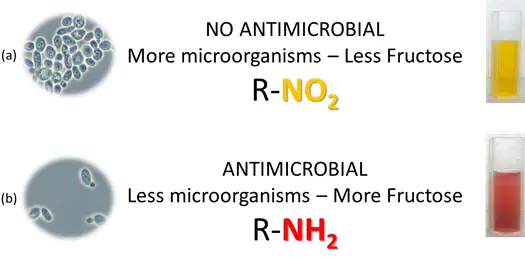
One of the main aims of this and prior research [9,10] was related to using a biochemical method that could provide reliable biocidal results for the different PVD-coating types. With that in mind, a rapid test for the evaluation of biocidal performance was developed that can rank different coating types for bioactivity within 1 to 3 days. This is a powerful means of speeding up coating development as results rapidly are available to link biocidal and coating properties. It also enables a quality-control check for production coatings to verify the reliability of the coating production.
The method was based on the titration of a reducing sugar, such as fructose, which is metabolized by a suitable microbe, such as yeast. The microbe needs to be representative of a bacteria responding to the same type of oxidative stresses that would produce bacteria-killing. It was found that the yeast Saccharomyces Cerevisiae was a suitable choice for the test, even though it is not a bacteria itself.
This quick test uses a colorimetric reaction called the Miller reaction (see Figure 8) [16]. The oxidation of the ketone functional group present in the reducing sugar – fructose, in this case – will favor the reduction of NO2 group of 3,5-dinitrosacylic acid (yellow) to NH2 resulting in 3-amino-5-nitro salicylic acid (red color solution). The color change can be quantitatively assessed, and a titration of the non-consumed fructose can be measured.
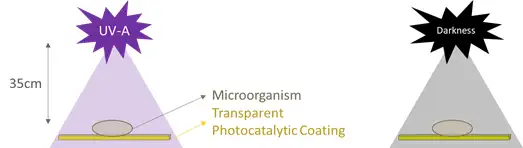
An appropriate data analysis and treatment are needed to produce a suitable scale that would reflect the biocidal activity of a given surface. The method was tested and calibrated against several antimicrobial standards and a biological test based on the Japanese Industrial Standard JIS Z 2801:2000 [17]. A surface with no biocidal effect represents 0% in the scale as it would consume all fructose, leaving a yellow color on the Miller’s reaction. For each test, the absorbance at 575 nm was placed with reference to the 0-100% scale.
The testing of the yeast solution on the surfaces in both light and darkness is used to assess effect of UV-light activation of the coating (see Figure 9).
Biocidal results of sputtered transparent layers
![Figure 10. The kill effectiveness of zinc and titanium oxide-based materials by biochemical test after UV-light reaction for 4 hrs. Blank samples (maximum of cfu/mL) and ZnOx samples with yellow solution. ZnOx coating have the lowest biocidal effect (less than Log 1 [90%] kill effect). This is compared with red solution coming from the Log 6 (99.9999%) kill effect) TiZnOx coatings developed, such as the active zero microbe (minimum of cfu/mL) to 100% kill effectiveness.](https://convertingquarterly.com/wp-content/uploads/2025/02/cq121monaghan_fig10-1.webp)
The method, developed by Gencoa, has created a simple scale for biocidal-surface efficacy by normalizing blank (maximum of cfu/mL) to 0% biocidal effect and a solution with zero microorganisms (minimum of cfu/mL) up to 100% kill effectiveness.
Different coatings have been deposited and tested to compare their suitability as transparent biocidal layers. Titanium dioxide is a well-known bioactive coating type that is activated by UV light to produce a photocatalytic effect. TiO2-based materials have been created with various alloy additions and compared to zinc-oxide-based layers (see Figure 10). The activation by UV light directly onto the sample confirms that such TiO2-based layers are highly biocidal, giving a Log 6 (99.9999%) kill effect after 4 hrs of yeast exposure on the surfaces (see Figure 10).
While titanium-oxide-based coatings are highly effective, the reliance upon UV light is a strong negative as this wavelength of light is not present in internal areas of buildings. As shown in Figure 11, the absence of UV light renders the same films completely inactive.
Transparent biocidal coatings which can operate in darkness (& light) will have much wider market appeal. Hence, experiments based upon transparent-copper compounds were conducted to identify a formula that could provide biocidal activity without the need for daylight. The aim was to combine the known copper-biocidal activity in a transparent form.
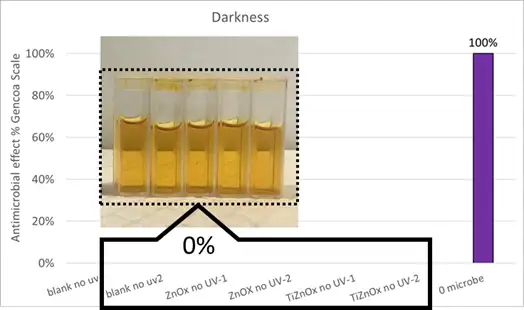
Figure 12 shows a range of results conducted in darkness with a 1-hr contact time for the yeast solution. This compares different forms of sputtered copper with a transparent-copper compound (green). The results show that such a highly biocidal, copper-based film can be created with a high degree of transparency which does not require UV-light activation. These transparent layers are as bioactive as optimized sputtered copper, and hence are extremely effective.
Discussion
Vacuum coating has the ability to create growth features on a nanometer scale from a wide range of material types. The growth features enhance the biocidal performance of vacuum-deposited materials when compared to a surface made by conventional methods. Copper is a well-known biocidal surface, and thermomechanically produced copper materials are not as effective as a vacuum-deposited copper. This is true for sputtered and evaporated vacuum-deposited copper which routinely have a LOG 5 or 6 kill rate compared to LOG 3 for conventional copper surfaces. Also, too “perfect” of a copper surface is not as effective as a rougher or oxidized surface.
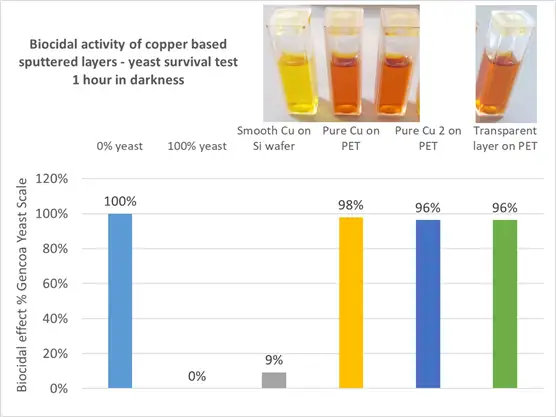
While copper coatings can offer protection, for visual touchscreen surfaces a transparent form is required (see Figure 13). Current forms of transparent biocidal materials are based upon titanium-oxide and require UV light for photocatalytic activation, hence, can be effective only in outside areas with strong natural daylight. A new copper compound has been discovered that can combine high transparency with very high levels of biocidal activity. This copper compound performs to a LOG 5-6 scale of killing activity in a short space of time. The reactive sputtering can be implemented readily on a large production scale to produce plastic- and glass-coated substrates.
Another strength of vacuum coating is the ability to reduce the amount of active ingredient, and hence the cost. Vacuum-coating thicknesses vary from a few nanometers to a few microns. For biocidal coatings, a few tens of nanometers are effective. Even thin alternative materials, such as copper tape, require 35 microns of metal. Other dip- or spray-coating processes produce coatings that are tens or 100s of microns thick. Film material that contains silver within the material will concentrate the active ingredient throughout the material stock, instead of at the surface where it is required. Hence vacuum coating always will “win” the economic race to minimize material use. A round-robin study of commercial alternatives is ongoing and shows that the rival technologies either are very mild compared to vacuum copper or offer little benefit over the base plastic performance.
The use of a “vacuum” environment adds cost compared to atmospheric processes, but this vacuum element is the key to creating and using very small amounts of materials on surfaces. As the vacuum-coating industry is mature, very high speed and efficient processing routes are widely available. In particular, R2R coating of plastic is conducted at a large scale at tens to 100s of mpm. Hence, by coating a plastic film with a biocidal layer, followed by an adhesive backing, a low-cost protective pad is created that can adhere to a touch surface. These pads create a self-sanitizing effect that will rapidly kill microbes and viruses. This is ideal in the fight against COVID-19 and HCAI because the manual cleaning methods are not required, and the technology acts 24/7, thus the probability of all forms of decease contagion is greatly reduced.
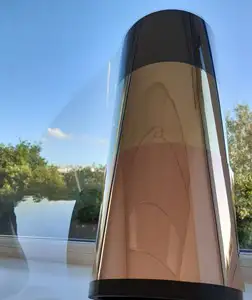
Perhaps the biggest challenge of the modern era relates to touchscreens. They are designed to encourage hand contact to the surfaces that multiple users will interact with continually. It’s hard to imagine a worse scenario when trying to tackle contagion. Voice-activated screens will be a very effective solution. But how reliable will that technology be in public places with background noise and different accents? Touchscreens are here to stay for the foreseeable future. As has been shown, there now is a very effective vacuum-coating solution to greatly reduce the risk of using such screens. The transparent film can adhere to existing screens to offer the same highly biocidal performance that vacuum-deposited copper can impart. As the layer activation is not light-dependent, it will have 24/7 operation and will last months / years as they have good abrasion resistance.
What are the downsides?
Clearly, if more plastic finds its way into the oceans, the public will have reservations about its eco-credentials. However, these are far from single-use items; at least six months of life is expected. There are a range of biodegradable plastic-film options, which will be assessed to ensure they can impart the same stable biocidal action over extended periods. Such self-sanitizing surfaces will greatly reduce the need for use of cleaning chemicals and their disposal, which is a positive factor.
The toxic effect of nanomaterials on humans is a concern in the health industry. Small <100-nm nanomaterials (particles) can cross biological membranes and access cells, tissues and organs that larger-sized particles normally cannot. However, this coated form of nanotechnology is designed to remain intact within the bulk coating and avoid breakdown or release from the underlying substrate. This assumption will need to be verified to avoid concerns related to nanoparticle generation. However, on a scale of risks compared to the negative impact of COVID-19 and HCAI, a rational approach would be encouraged to prevent regulatory barriers for adoption. Copper-based materials have the advantage of US FDA approval.
Conclusions
This work has successfully created highly biocidal, sputtered coatings based upon copper. The sputtered copper performs better than conventional copper materials moving the surface bioactivity from LOG 3 to LOG 6. A reliable method for quantifying surfaces’ biocidal activity has been developed to accurately rank different layer types. Based on the rapid testing capability, it has been possible to create a new form of transparent coating that does not rely upon UV light for activation. The copper-based compound kills microbes and viruses at the same speed as sputtered copper and will afford the same round-the-clock, self-sanitizing effect. The broad-spectrum, biological-killing capacity that these coatings provide combined with low cost of production should drive widespread adoption. Sputter PVD can create a “super” surface of chemical delivery and morphology in a highly robust form with an attractive appearance.
The final piece of the puzzle remains unanswered: How quickly is the SARS-CoV-2 virus killed on these surfaces? It is estimated that the half-life will be less than 5 mins, and testing will be conducted in the next few months to quantify the killing speed. A new test method at AIN in Spain to assess how effective surfaces are at viral killing has been used on these sputtered-copper surfaces. The test sample is put in contact with solution containing 1 million viral particles [Coronavirus TGEV Transmissible Gastroenteritis Coronavirus (BSL-2)]. After just 15 mins of contact, no viable particles remained. This confirms the effectiveness against viruses on surfaces and also will mean that flu and the common cold will be unable to survive on such surfaces for more than a few minutes. Even once the COVID-19 pandemic passes, there remains the problem to society of Healthcare Acquired Infection (HCAI) and the potential of future pandemics. “Don’t touch that surface unless it is sputtered” could become the choice to avoid future decease contagion.
Acknowledgements
The authors would like to thank Joseba Esparza-Gorraiz, José Fernández-Palacios and Gonzalo Garcia-Fuentes of AIN Surface Technology Group (Asociación de la Industria Navarra, Spain, www.ain.es/tecnologia/superficies/) for their assistance with the antimicrobial JIS Z 2801:2000 and viral test. The Open Innovation Hub for Antimicrobial Surfaces at the University of Liverpool, UK. Innovate UK project 103820 (2017), 78311, 85336, 85562 (2020). Erasmus Project nº 2019-1-ES01-KA103-063357.
References
9. “High-Efficiency Antimicrobial Coatings for Biomedical Applications.” Authors: Oihane Hernandez-Rodriguez, Victor Bellido-Gonzalez, Dermot Monaghan Published: 2018 Society of Vacuum Coaters TechCon.
10. “Antimicrobial Coatings for Touch Surfaces.” Authors: Oihane Hernandez-Rodriguez, Dermot Monaghan, Victor Bellido-Gonzalez. Published: 2019 Society of Vacuum Coaters TechCon.
14. V. Bellido-Gonzalez, et al. “Long-term Process Control and Stability in reactive Sputtering,” SVC 2005, 505/856-7188.
15. D. Monaghan, et al. “Using a Standard Penning Gauge as a Powerful Means of Monitoring and Feedback Control,” SVC 2007, 505/856-7188.
16. G. L. Miller; Analytical Chemistry, 1959, 31 (3), pp 426-428.
17. Japanese Industrial Standard Committee (2000) “Antimicrobial products: Test for antimicrobial activity and efficacy,” Publication JIS Z 2801:2000. Japanese Standards Assn., Tokyo.
Dr. Dermot Monaghan of Gencoa, Ltd. (Liverpool, UK), can be reached at +44-151-486-4466, direct: +44-151-541-6030, email: [email protected] or www.gencoa.com.
Oihane Hernandez-Rodriguez of Fundación Tekniker (Eibar, Spain), can be reached at +34-943-20-67-44 or https://www.tekniker.es/en.